Een nieuw ontwerp van een intelligent medicijnafgiftesysteem op basis van nanoantennedeeltjes
Abstract
Geneesmiddelafgiftesysteem voor samengestelde nanodeeltjes speelt een belangrijke rol in de interactie met lymfeklieren. Er zijn drie primaire typen lymfocyten:B-cellen, T-cellen en natuurlijke killercellen. Wanneer de cellen van het immuunsysteem kankerverwekkend worden, vallen ze lichaamscellen aan. Het lymfevocht speelt een belangrijke rol bij het aanvallen van gezonde lichaamscellen; daarom was dit artikel bedoeld om een medicijnafgiftesysteem te ontwerpen, dat nanodeeltjes efficiënt kan sturen om de geïnfecteerde cellen te targeten, wat helpt bij de snelle eliminatie van dergelijke cellen. Het voorgestelde ontwerp hangt af van de interactie tussen deze moleculen en de intelligente nano-controller heeft het vermogen om de nanodeeltjes te sturen door anaëroob contact. Het voorgestelde ontwerp bewees dat hoe kleiner de nanodeeltjesgrootte en -dichtheid, hoe minder dynamische viscositeit van de vloeistof zou zijn, wat de weerstand tegen stroming zou weerspiegelen. Bovendien werd geconcludeerd dat waterstofmoleculen een belangrijke rol spelen bij het verminderen van de weerstand van lymfevocht vanwege hun lage dichtheid.
Inleiding
De huidige behandelingsopties voor kanker omvatten chirurgie, bestraling en chemotherapie. Deze behandelingsstrategieën beschadigen ook gewone weefsels en resulteren in gedeeltelijke vernietiging van kwaadaardige groei. Daarom kan nanotechnologie deze tekortkomingen verhelpen door zich specifiek op schadelijke cellen en neoplasma te richten, tumoren direct te verwijderen en de effectiviteit van op straling gebaseerde en andere behandelingsmodaliteiten te vergroten. Dit kan de nadelige effecten van de behandeling aanzienlijk verminderen en de overlevingskans verhogen. Nanotechnologie is een veelbelovend hulpmiddel voor de behandeling van kwaadaardige groei omdat het nieuwere en betere behandelingsmodaliteiten biedt door gebruik te maken van nanomateriaal. Nanodeeltjes kunnen zich specifiek richten op veel moleculen die differentieel tot expressie worden gebracht op kankercellen. Het over het algemeen enorme aërodynamische gebied van nanodeeltjes kan worden gefunctionaliseerd met liganden zoals kleine deeltjes en deoxyribonucleic corrosieve of ribonucleic corrosieve keten peptide-antilichamen. De liganden worden gebruikt als medicijn en in theranostische toepassingen. De fysieke eigenschappen van nanodeeltjes, zoals afleiding en herbestraling van vitaliteit, kunnen ook worden gebruikt om ziek weefsel aan te tasten, zoals bij laserverwijdering en hyperthermie-toepassingen [1].
Het innovatieve softwareprogramma voor nanodeeltjes en het actieve farmaceutische element zullen ook de verkenning van een breder repertoire van actieve ingrediënten mogelijk maken. Daarom worden de immunogene lading en oppervlaktecoating onderzocht als beide adjuvantia voor door nanodeeltjes gemedieerde en traditionele chemotherapie. Deze innovatieve strategie omvat het ontwerp van nanodeeltjes als kunstmatig antigeen dat op cellen wordt gepresenteerd en in vivo depots van stimulerende factoren die antitumoreffecten uitoefenen. De nanotechnologie vertegenwoordigt een actief onderzoeksgebied met veel toepassingen. Nanodeeltjes hebben interesse gekregen in medische technologie vanwege hun afstembare fysisch-chemische eigenschappen zoals dooiindexpunt, hydrofiliciteit, elektrische en thermische geleiding, katalytische activiteit, lichtabsorptie en verstrooiing [2]. In principe worden nanomaterialen beschreven als materialen met deeltjes in het bereik van 1 tot 100 nm. Er zijn verschillende wetgevingen in de Europese Unie en de VS die specifiek verwijzen naar medisch onderzoek met nanomaterialen. Er is echter geen internationaal aanvaarde definitie van nanomaterialen. Verschillende organisaties beschouwen verschillende concepten van nanomaterialen [3]. Een van de doelen van het medicijnafgiftesysteem voor nanodeeltjes is het behandelen van lymfevocht met kankercellen. Een samengesteld medicijnafgiftesysteem met nanodeeltjes in interactie met lymfeklieren wordt getoond in figuur 1.
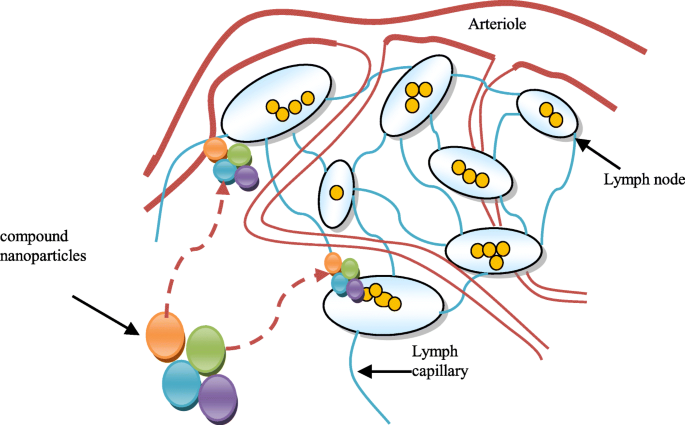
Samengesteld medicijnafgiftesysteem voor nanodeeltjes en de interactie ervan met lymfeklieren
De Amerikaanse Food and Drug Administration verwijst naar nanomaterialen als materialen met deeltjes in het bereik van 1 tot 100 met andere eigenschappen dan het bulkmateriaal [4, 5]. Nanovezels, nanoplaten, nanodraden, kwantumdots en andere verwante materialen zijn gekarakteriseerd [6]. Vaste lipide-nanodeeltjes (SLN's) zijn een soort lipide-nanodeeltjes (LN), die kunnen worden geconstrueerd door gebruik te maken van vaste lipiden [7]. Latere versies van SLN's zijn ontwikkeld, zoals nanogestructureerde lipidedragers (NLC's), die het tweede tijdperk van LN vertegenwoordigen [8]. Zowel SLN als NLC zijn opgebouwd uit vaste lipiden. De inwendige structuur van SLN bevat vaste lipiden, terwijl NLC is ontwikkeld met behulp van een mengsel van vaste en vloeibare lipiden, die een dwarsdoorsnede van edelstenen produceren [9, 10]. Deze tekortkomingen zijn bovendien gemeld voor SLN's in het licht van het feit dat SLN's die veel vaste lipidesegmenten bevatten, kunnen worden gebruikt in medische toepassingen [11, 12]. Polymere nanodeeltjes (PN) kunnen worden opgebouwd uit natuurlijke polymeren of anorganische materialen, bijvoorbeeld silica [13]. De polymeren of lipiden vormen de kern van NP's, die de stabiliteit en medicijnafgifte verbeteren en een uniforme vorm en grootte bieden [14]. PN kan worden beschreven als nanocapsules of nanosferen. De nanocapsules bevatten olie in een blaasjesstructuur samen met een medicijn [15, 16], terwijl nanosferen polymere ketens bevatten zonder olie [17, 18]. Een medicijn wordt verpakt in PN's door vermenging met het polymeer. De opname van het medicijn is verzekerd in de nanodeeltjes op het moment van polymerisatie. PN's worden geladen met een medicijn door het op te lossen, te verstrooien of kunstmatig te adsorberen in de bestanddelen van het polymeernetwerk [19, 20]. Er zijn drie soorten lymfocyten:B-cellen, T-cellen en natuurlijke killercellen. De B-cellen maken antilichamen aan die binnendringende micro-organismen aanvallen, terwijl ze ook het immuunsysteem aanvallen wanneer ze kankerverwekkend worden. Gezien de belangrijke rol van lymfevocht bij auto-immuniteit, was het doel van dit artikel daarom het ontwerpen van een intelligent medicijnafgiftesysteem op basis van nanoantennedeeltjes. Het systeem bevat dus veel nanodeeltjes in verschillende hoeveelheden. In het volgende gedeelte wordt het ontwerp van een intelligent medicijnafgiftesysteem gepresenteerd.
Ontwerp van een nano-intelligent medicijnafgiftesysteem
Het voorgestelde nano-intelligente medicijnafgiftesysteem bevat een nanocontroller die wordt aangedreven door een elektrische bron van nanodeeltjes gemaakt van een nano-piëzo-elektrisch materiaal. De complexe opslagplaats van nanodeeltjes heeft een aantal microopslagplaatsen. Elke kleine repository bevat één type nanodeeltjes. Een nanodeeltjesmolecuul bevat een nanoantenne die is ontworpen om te communiceren met de nanocontroller. Het voorgestelde nano-intelligente medicijnafgiftesysteem bevat ook koolstofnanobuisjes voor de snelle levering van medicijnen aan kankercellen. Het kan worden geassocieerd met de geïnfecteerde cellen, zoals weergegeven in figuur 2. Het systeem begint met het sturen van nanodeeltjes naar de kankercellen die 'verkennende nanodeeltjes' worden genoemd. Deze moleculen sturen door middel van anaërobe communicatie het complete beeld van hun positie in de cellen naar de nanocontroller. Op basis van de situatie die de verkennende nanodeeltjes tegenkomen, stuurt de nanocontroller nanodeeltjes van verschillende aantallen, soorten en dichtheid naar de kankercellen, op basis van de informatie die is verzameld uit de verkennende nanodeeltjes. Deze nanodeeltjes worden "vechtende nanodeeltjes" genoemd.
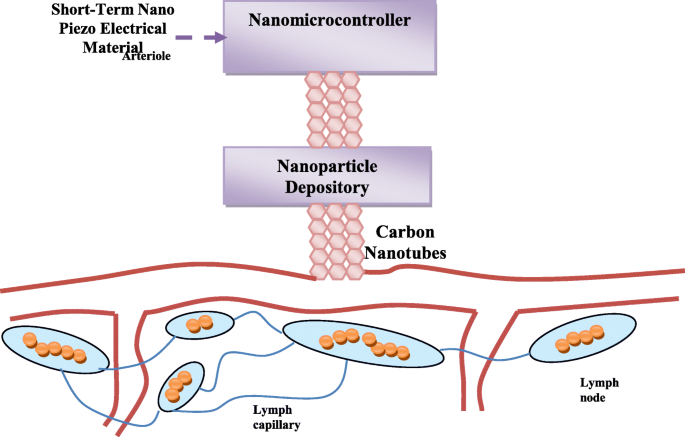
Algemene structuur die de associatie van het voorgestelde medicijnsysteem met de geïnfecteerde cellen toont
Dit is geen willekeurig proces, maar wordt gecontroleerd door de nano-controller, rekening houdend met verschillende aspecten en logaritmen, die een efficiënte en snelle levering van de nanodeeltjes zullen garanderen. Om de nanodeeltjes nauwkeurig en snel aan de kankercellen te leveren, zal het compressieve binaire zoekalgoritme worden gebruikt [21]. Verder zullen nanodeeltjes in verschillende dichtheden worden afgeleverd, zodat het medicijn effectiever wordt. Deze methodologieën en hun modus operandi door gebruik te maken van de nano-controller worden geïllustreerd in figuur 3. De fysieke structuur van de nano-controller is vergelijkbaar met die van de nanodeeltjes, maar heeft de vorm van metaal zodat het elektrische energie kan winnen voor een korte periode tijdens het werken. Dit metaal bevat een draadloze antenne samen met een klein geheugen dat de bedieningscodes bevat met een nanodeeltjeslink tussen de nanocontroller en de nanodeeltjesopslag. De opslagplaats voor nanodeeltjes bevat verschillende soorten nanodeeltjes. Het openen en sluiten, evenals de duur van het openen van de nano-poort, worden gecontroleerd om het aantal af te leveren deeltjes aan te passen.
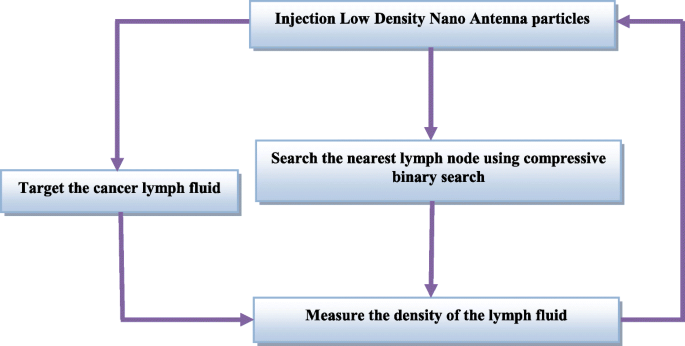
Verzenden van de vechtende nanodeeltjes naar kankercellen
Een beschrijving van de aard van de nanodeeltjes die in het voorgestelde geneesmiddelensysteem worden gebruikt
In de volgende sectie wordt de aard van de nanodeeltjes die in het voorgestelde medicijnafgiftesysteem worden gebruikt, besproken. In dit werk werden anaërobe nanodeeltjes met een lage dichtheid gebruikt zoals beschreven in een eerder rapport [22].
Nanodeeltjes met lage dichtheid
Beschouw het medicijnafgifteproces van samengestelde nanodeeltjes voor kanker als een penetrerend proces in lymfevocht, waar een tumor wordt omgeven door het lymfevocht. De samenstelling van het melanoom lijkt op het lymfevocht. Het voorgestelde analytische model is gebaseerd op een nanobuissysteem dat bestaat uit drie verschillende soorten nanodeeltjes. De nanodeeltjes worden in een lymfevloeistof met hoge dichtheid geplaatst. We kunnen specifieke nanodeeltjes van vast A in de sferische poolcoördinaten definiëren als A = (ra, ϑa , φa), waarbij ra de radiale coördinaat is voor het nanodeeltje van vast A, ϑa is de zenitale coördinaat voor het nanodeeltje van vast A, en φa is de azimutale coördinaat voor het nanodeeltje van vast A. De corresponderende coördinaten van vast B zijn B = (rb, ϑb, φb), respectievelijk, en de corresponderende coördinaten van vast N zijn N = (rn, ϑn, φn), respectievelijk. Bedenk dat er twee eigenschappen van de lymfeklier zijn, namelijk zacht en gezwollen, die worden beïnvloed door de kankercellen van Hodgkin-lymfoom. De lymfeklier met gevoelige eigenschap Tp kan worden omschreven als Tp (N ,t ); dit betekent dat de waarde van Tp in samenhang met de vloeistof van nanodeeltjes van vast N met de tijd varieert. Laten we nu bedenken dat het totale effect van de samengestelde nanodeeltjes in de aanbestedingseigenschap wordt gedefinieerd als:
$$ \mathrm{Tpt}=\mathrm{Tp}\ \left(A,t\right)+\mathrm{Tp}\ \left(B,t\right)+.\dots \dots \dots \dots + \mathrm{Tp}\ \links(N,t\rechts) $$ (1)Overweeg hetzelfde geval voor de gezwollen eigenschap, die kan worden gedefinieerd als:
$$ \mathrm{Tst}=\mathrm{Ts}\ \left(A,t\right)+\mathrm{Ts}\ \left(B,t\right)+.\dots \dots \dots \dots + \mathrm{Ts}\ \links(N,t\rechts) $$ (2)Van eq. 1 en 2, kan de veranderingssnelheid van beide eigenschappen in de tijd worden bepaald als:
$$ \frac{\partial \left(\mathrm{Tp}\left(A,t\right)\right)}{\partial t}+\frac{\partial \left(\mathrm{Tp}\left( B,t\right)\right)}{\partial t}+\dots \frac{\partial \left(\mathrm{Tp}\left(N,t\right)\right)}{\partial t}=\frac{\mathrm{\partial Tp}(t)}{\mathrm{\partial t}} $$ (3) $$ \frac{\partial \left(\mathrm{Ts}\left(A,t\ right)\right)}{\partial t}+\frac{\partial \left(\mathrm{Ts}\left(B,t\right)\right)}{\partial t}+\dots \frac{\ gedeeltelijk \left(\mathrm{Ts}\left(N,t\right)\right)}{\partial t}=\frac{\mathrm{\partial Ts}(t)}{\partial t} $$ ( 4)Het punt in de lymfevloeistof dat kan worden ingenomen door één nanodeeltje vast N wordt gedefinieerd als:
$$ {\mathrm{Po}}_n=\mathrm{Po}{\left(\mathrm{po},t\right)}_n $$ (5)Laten we eens kijken naar een nanodeeltje van het vaste N-derivaat van de zachte lymfevloeistof wordt gedefinieerd als \( \frac{\partial {\left(\mathrm{Tp}\left(N,t\right)\right)}_{\ mathrm{po}}}{\partial t} \), dan zou het samengestelde materiaalderivaat van de gevoelige lymfevloeistof gelijk zijn aan:
$$ \frac{\partial {\left(\mathrm{Tp}\left(A,t\right)\right)}_{\mathrm{po}}}{\partial t}+\frac{\partial { \left(\mathrm{Tp}\left(B,t\right)\right)}_{\mathrm{po}}}{\partial t}+\dots \frac{\partial {\left(\mathrm{ Tp}\left(N,t\right)\right)}_{\mathrm{po}}}{\partial t}=\frac{\mathrm{\partial Tp}{(t)}_{\mathrm{ po}}}{\partial t} $$ (6) $$ \frac{\partial {\left(\mathrm{Ts}\left(A,t\right)\right)}_{\mathrm{po} }}{\partial t}+\frac{\partial {\left(\mathrm{Ts}\left(B,t\right)\right)}_{\mathrm{po}}}{\partial t}+ \dots \frac{\partial {\left(\mathrm{Ts}\left(N,t\right)\right)}_{\mathrm{po}}}{\partial t}=\frac{\mathrm{ \partial Ts}{(t)}_{\mathrm{po}}}{\partial t} $$ (7)De corresponderende snelheidscomponenten van de vaste N worden genomen als (v rn , v ϑn , v φn ). Vervolgens wordt de stroomsnelheid van de deeltjes van vast N weergegeven met behulp van Navier-Stokes-vergelijkingen bij dynamische viscositeit dν van de lymfevloeistof, en p is de druk en ρ is de dichtheid van lymfevocht als volgt:
$$ \frac{\partial {v}_{\mathrm{rn}}}{\partial t}+{v}_{\mathrm{rn}}\frac{\partial {v}_{\mathrm{rn }}}{\mathrm{\partial rn}}+\frac{v_{\upvartheta \mathrm{n}}}{\mathrm{rn}}\frac{\partial {v}_{\mathrm{rn}} }{\mathrm{\partial \upvartheta n}}+\frac{v_{\upvarphi \mathrm{n}}}{\mathrm{rn}\ \mathrm{sin}\upvartheta \mathrm{n}}\frac{ \partial {v}_{\mathrm{rn}}}{\mathrm{\partial \upvarphi n}}-\frac{v_{\upvartheta \mathrm{n}}^2}{\mathrm{rn}}- \frac{v_{\upvarphi \mathrm{n}}^2}{\mathrm{rn}}+\frac{1}{\rho}\frac{\partial p}{\mathrm{\partial rn}}- \mathrm{d}\upnu \left[\frac{1}{{\mathrm{rn}}^2}\frac{\partial}{\mathrm{\partial rn}}\left({\mathrm{rn} }^2\frac{\partial {v}_{\mathrm{rn}}}{\mathrm{\partial rn}}\right)+\frac{1}{{\mathrm{rn}}^2\mathrm {sin}\upvartheta \mathrm{n}}\frac{\partial }{\mathrm{\partial \upvartheta n}}\left(\mathrm{sin}\upvartheta \mathrm{n}\frac{\partial {v }_{\mathrm{rn}}}{\mathrm{\partial \upvartheta n}}\right)+\frac{1}{{\mathrm{rn}}^2{\sin}^2\upvartheta \mathrm {n}}\frac{\partial^2{v}_{\ma thrm{rn}}}{\partial {\upvarphi \mathrm{n}}^2}+-\frac{2{v}_{\mathrm{rn}}}{{\mathrm{rn}}^2} -\frac{2}{{\mathrm{rn}}^2\sin \upvartheta \mathrm{n}}\frac{\partial \left({v}_{\upvartheta \mathrm{n}}\mathrm{ sin}\upvartheta \mathrm{n}\right)}{\mathrm{\partial \upvartheta n}}-\frac{2}{{\mathrm{rn}}^2\mathrm{sin}\upvartheta \mathrm{ n}}\frac{\partial {v}_{\upvarphi \mathrm{n}}}{\partial_{\upvarphi \mathrm{n}}}\right]=0 $$ (8)De dynamische viscositeit dν van het lymfevocht wordt als volgt berekend:
$$ \mathrm{d}\upnu =\frac{\left[\frac{\partial {v}_{\mathrm{rn}}}{\partial t}+{v}_{\mathrm{rn}} \frac{\partial {v}_{\mathrm{rn}}}{\mathrm{\partial rn}}+\frac{v_{\upvartheta \mathrm{n}}\partial {v}_{\mathrm{ rn}}}{\mathrm{rn}\ \mathrm{\partial \upvartheta n}}+\frac{v_{\upvarphi \mathrm{n}}}{\mathrm{rn}\ \mathrm{sin}\upvartheta \mathrm{n}}\frac{\partial {v}_{\mathrm{rn}}}{\mathrm{\partial \upvarphi n}}-\frac{v_{\upvartheta \mathrm{n}}^2 }{\mathrm{rn}}-\frac{v_{\upvarphi \mathrm{n}}^2}{\mathrm{rn}}+\frac{1\ \partial p}{\uprho \partial \mathrm{ rn}}\right]}{\left[\frac{1}{{\mathrm{rn}}^2}\frac{\partial }{\mathrm{\partial rn}}\left({\mathrm{rn }}^2\frac{\partial {v}_{\mathrm{rn}}}{\mathrm{\partial rn}}\right)+\frac{1}{{\mathrm{rn}}^2\ mathrm{sin}\upvartheta \mathrm{n}}\frac{\partial }{\mathrm{\partial \upvartheta n}}\left(\mathrm{sin}\upvartheta \mathrm{n}\frac{\partial { v}_{\mathrm{rn}}}{\mathrm{\partial \upvartheta n}}\right)+\frac{1}{{\mathrm{rn}}^2{\sin}^2\upvartheta \ mathrm{n}}\frac{\partial ^2{v}_{\mathrm{rn}}}{\partial {\upvarphi \mathrm{n}}^2}+-\frac{2{v}_{\mathrm{rn}}}{{\ mathrm{rn}}^2}-\frac{2}{{\mathrm{rn}}^2\sin \upvartheta \mathrm{n}}\frac{\partial \left({v}_{\upvartheta \ mathrm{n}}\mathrm{sin}\upvartheta \mathrm{n}\right)}{\mathrm{\partial \upvartheta n}}-\frac{2}{{\mathrm{rn}}^2\mathrm {sin}\upvartheta \mathrm{n}}\frac{\partial {v}_{\upvarphi \mathrm{n}}}{\partial_{\upvarphi \mathrm{n}}}\right]} $$ ( 9)De Navier-Stokes-vergelijkingen van vaste stoffen A en B kunnen worden weergegeven als Vgl. 8 en 9. Dus, Vgl. 9 kan als volgt worden weergegeven:
$$ \mathrm{d}\upnu =\frac{\left[\frac{\partial {v}_{\mathrm{rn}}}{\partial t}+{v}_{\mathrm{rn}} \frac{\partial {v}_{\mathrm{rn}}}{\mathrm{\partial rn}}+\frac{v_{\upvartheta \mathrm{n}}}{\mathrm{rn}}\frac {\partial {v}_{\mathrm{rn}}}{\mathrm{\partial \upvartheta n}}+\frac{v_{\upvarphi \mathrm{n}}}{\mathrm{rn}\ \mathrm {sin}\upvartheta \mathrm{n}}\frac{\partial {v}_{\mathrm{rn}}}{\mathrm{\partial \upvarphi n}}-\frac{v_{\upvartheta \mathrm{ n}}^2}{\mathrm{rn}}-\frac{v_{\upvarphi \mathrm{n}}^2}{\mathrm{rn}}+\frac{1}{\rho}\frac{ \partial p}{\mathrm{\partial rn}}\right]}{\left[\frac{1}{{\mathrm{rn}}^2}\frac{\partial}{\mathrm{\partial rn }}\left({\mathrm{rn}}^2\frac{\partial {v}_{\mathrm{rn}}}{\mathrm{\partial rn}}\right)+\frac{1}{ {\mathrm{rn}}^2\mathrm{sin}\upvartheta \mathrm{n}}\frac{\partial}{\mathrm{\partial \upvartheta n}}\left(\mathrm{sin}\upvartheta \ mathrm{n}\frac{\partial {\mathrm{v}}_{\mathrm{rn}}}{\mathrm{\partial \upvartheta n}}\right)+\frac{1}{{\mathrm{ rn}}^2{\sin}^2\upvartheta \math rm{n}}\frac{\partial^2{\mathrm{v}}_{\mathrm{rn}}}{\partial {\upvarphi \mathrm{n}}^2}-\frac{2{\ mathrm{v}}_{\mathrm{rn}}}{{\mathrm{rn}}^2}-\frac{2}{{\mathrm{rn}}^2\sin \upvartheta \mathrm{n} }\frac{\partial \left({\mathrm{v}}_{\upvartheta \mathrm{n}}\mathrm{sin}\upvartheta \mathrm{n}\right)}{\mathrm{\partial \upvartheta n}}-\frac{2}{{\mathrm{rn}}^2\mathrm{sin}\upvartheta \mathrm{n}}\frac{\partial {\mathrm{v}}_{\upvarphi \mathrm {n}}}{\partial_{\upvarphi \mathrm{n}}}\right]}=\frac{\left[\frac{\partial {\mathrm{v}}_{\mathrm{ra}}} {\mathrm{\partial t}}+{\mathrm{v}}_{\mathrm{ra}}\frac{\partial {\mathrm{v}}_{\mathrm{ra}}}{\mathrm{ \partial ra}}+\frac{{\mathrm{v}}_{\upvartheta \mathrm{a}}}{\mathrm{ra}}\frac{\partial {\mathrm{v}}_{\mathrm {ra}}}{\mathrm{\partial \upvartheta a}}+\frac{{\mathrm{v}}_{\upvarphi \mathrm{a}}}{\mathrm{ra}\ \mathrm{sin} \upvartheta \mathrm{a}}\frac{\partial {\mathrm{v}}_{\mathrm{ra}}}{\mathrm{\partial \upvarphi a}}-\frac{{\mathrm{v} }_{\upvartheta \mathrm{a}}^2}{\mathrm{ra}}- \frac{{\mathrm{v}}_{\upvarphi \mathrm{a}}^2}{\mathrm{ra}}+\frac{1}{\uprho}\frac{\mathrm{\partial p} }{\mathrm{\partial ra}}\right]}{\left[\frac{1}{{\mathrm{ra}}^2}\frac{\partial}{\mathrm{\partial ra}}\ left({\mathrm{ra}}^2\frac{\partial {v}_{\mathrm{ra}}}{\mathrm{\partial ra}}\right)+\frac{1}{{\mathrm {ra}}^2\mathrm{sin}\upvartheta \mathrm{a}}\frac{\partial}{\mathrm{\partial \upvartheta a}}\left(\mathrm{sin}\upvartheta \mathrm{a }\frac{\partial {v}_{\mathrm{ra}}}{\mathrm{\partial \upvartheta a}}\right)+\frac{1}{{\mathrm{ra}}^2{\ sin}^2\upvartheta \mathrm{a}}\frac{\partial^2{v}_{\mathrm{ra}}}{\partial {\upvarphi \mathrm{a}}^2}-\frac{ 2{v}_{\mathrm{ra}}}{{\mathrm{ra}}^2}-\frac{2}{{\mathrm{ra}}^2\sin \upvartheta \mathrm{a}} \frac{\partial \left({v}_{\upvartheta \mathrm{a}}\mathrm{sin}\upvartheta \mathrm{a}\right)}{\mathrm{\partial \upvartheta a}}-\ frac{2}{{\mathrm{ra}}^2\mathrm{sin}\upvartheta \mathrm{a}}\frac{\partial {v}_{\upvarphi \mathrm{a}}}{\partial_{ \upvarphi \mathrm{a}}}\right]}=\frac{ \left[\frac{\partial {v}_{\mathrm{rb}}}{\partial t}+{v}_{\mathrm{rb}}\frac{\partial {v}_{\mathrm{ rb}}}{\mathrm{\partial rb}}+\frac{v_{\upvartheta \mathrm{b}}}{\mathrm{rb}}\frac{\partial {v}_{\mathrm{rb} }}{\mathrm{\partial \upvartheta b}}+\frac{v_{\upvarphi \mathrm{b}}}{\mathrm{rb}\ \mathrm{sin}\upvartheta \mathrm{b}}\frac {\partial {v}_{\mathrm{rb}}}{\mathrm{\partial \upvarphi b}}-\frac{v_{\upvartheta \mathrm{b}}^2}{\mathrm{rb}} -\frac{v_{\upvarphi \mathrm{b}}^2}{\mathrm{rb}}+\frac{1}{\rho}\frac{\partial p}{\mathrm{\partial rb}} \right]}{\left[\frac{1}{{\mathrm{rb}}^2}\frac{\partial }{\mathrm{\partial rb}}\left({\mathrm{rb}}^ 2\frac{\partial {v}_{\mathrm{rb}}}{\mathrm{\partial rb}}\right)+\frac{1}{{\mathrm{rb}}^2\mathrm{sin }\upvartheta \mathrm{b}}\frac{\partial }{\mathrm{\partial \upvartheta b}}\left(\mathrm{sin}\upvartheta \mathrm{b}\frac{\partial {v}_ {\mathrm{rb}}}{\mathrm{\partial \upvartheta b}}\right)+\frac{1}{{\mathrm{rb}}^2{\sin}^2\upvartheta \mathrm{b }}\frac{\partial^2{v}_{\mathrm{ rb}}}{\partial {\upvarphi \mathrm{b}}^2}-\frac{2{v}_{\mathrm{rb}}}{{\mathrm{rb}}^2}-\frac {2}{{\mathrm{rb}}^2\sin \upvartheta \mathrm{b}}\frac{\partial \left({v}_{\upvartheta \mathrm{b}}\mathrm{sin}\ upvartheta \mathrm{b}\right)}{\mathrm{\partial \upvartheta b}}-\frac{2}{{\mathrm{rb}}^2\mathrm{sin}\upvartheta \mathrm{b}} \frac{\partial {v}_{\upvarphi \mathrm{b}}}{\partial_{\upvarphi \mathrm{b}}}\right]} $$ (10)De deeltjes hebben nano-dimensies; dus zouden hun stralen erg klein zijn, en voor de eenvoud zou Vgl. 10 wordt als volgt weergegeven:
$$ \mathrm{d}\upnu =\left[\frac{v_{\upvartheta \mathrm{n}}}{\mathrm{rn}}\frac{\partial {v}_{\mathrm{rn}} }{\mathrm{\partial \upvartheta n}}+\frac{v_{\upvarphi \mathrm{n}}}{\mathrm{rn}\ \mathrm{sin}\upvartheta \mathrm{n}}\frac{ \partial {v}_{\mathrm{rn}}}{\mathrm{\partial \upvarphi n}}-\frac{v_{\upvartheta \mathrm{n}}^2}{\mathrm{rn}}- \frac{v_{\upvarphi \mathrm{n}}^2}{\mathrm{rn}}+\frac{1}{\rho}\frac{\partial p}{\mathrm{\partial rn}}\ right]/\left[\frac{1}{{\mathrm{rn}}^2}\frac{\partial }{\mathrm{\partial rn}}\left({\mathrm{rn}}^2\ frac{\partial {v}_{\mathrm{rn}}}{\mathrm{\partial rn}}\right)+\frac{1}{{\mathrm{rn}}^2\mathrm{sin}\ upvartheta \mathrm{n}}\frac{\partial }{\mathrm{\partial \upvartheta n}}\left(\mathrm{sin}\upvartheta \mathrm{n}\frac{\partial {\mathrm{v} }_{\mathrm{rn}}}{\mathrm{\partial \upvartheta n}}\right)+\frac{1}{{\mathrm{rn}}^2{\sin}^2\upvartheta \mathrm {n}}\frac{\partial^2{\mathrm{v}}_{\mathrm{rn}}}{\partial {\upvarphi \mathrm{n}}^2}-\frac{2{\mathrm {v}}_{\mathrm{rn}}}{{\math rm{rn}}^2}-\frac{2}{{\mathrm{rn}}^2\sin \upvartheta \mathrm{n}}\frac{\partial \left({\mathrm{v}}_ {\upvartheta \mathrm{n}}\mathrm{sin}\upvartheta \mathrm{n}\right)}{\mathrm{\partial \upvartheta n}}-\frac{2}{{\mathrm{rn}} ^2\mathrm{sin}\upvartheta \mathrm{n}}\frac{\partial {\mathrm{v}}_{\upvarphi \mathrm{n}}}{\partial_{\upvarphi \mathrm{n}} }\right]=\left[\frac{{\mathrm{v}}_{\upvartheta \mathrm{a}}}{\mathrm{ra}}\frac{\partial {\mathrm{v}}_{ \mathrm{ra}}}{\mathrm{\partial \upvartheta a}}+\frac{{\mathrm{v}}_{\upvarphi \mathrm{a}}}{\mathrm{ra}\ \mathrm{ sin}\upvartheta \mathrm{a}}\frac{\partial {\mathrm{v}}_{\mathrm{ra}}}{\mathrm{\partial \upvarphi a}}-\frac{{\mathrm{ v}}_{\upvartheta \mathrm{a}}^2}{\mathrm{ra}}-\frac{{\mathrm{v}}_{\upvarphi \mathrm{a}}^2}{\mathrm {ra}}+\frac{1}{\uprho}\frac{\mathrm{\partial p}}{\mathrm{\partial ra}}\right]/\left[\frac{1}{{\mathrm {ra}}^2}\frac{\partial}{\mathrm{\partial ra}}\left({\mathrm{ra}}^2\frac{\partial {\mathrm{v}}_{\mathrm {ra}}}{\mathrm{\partial ra}}\rig ht)+\frac{1}{{\mathrm{ra}}^2\mathrm{sin}\upvartheta \mathrm{a}}\frac{\partial}{\mathrm{\partial \upvartheta a}}\left (\mathrm{sin}\upvartheta \mathrm{a}\frac{\partial {\mathrm{v}}_{\mathrm{ra}}}{\mathrm{\partial \upvartheta a}}\right)+\ frac{1}{{\mathrm{ra}}^2{\sin}^2\upvartheta \mathrm{a}}\frac{\partial^2{\mathrm{v}}_{\mathrm{ra}} }{\partial {\upvarphi \mathrm{a}}^2}-\frac{2{\mathrm{v}}_{\mathrm{ra}}}{{\mathrm{ra}}^2}-\ frac{2}{{\mathrm{ra}}^2\sin \upvartheta \mathrm{a}}\frac{\partial \left({\mathrm{v}}_{\upvartheta \mathrm{a}}\ mathrm{sin}\upvartheta \mathrm{a}\right)}{\mathrm{\partial \upvartheta a}}-\frac{2}{{\mathrm{ra}}^2\mathrm{sin}\upvartheta \ mathrm{a}}\frac{\partial {v}_{\upvarphi \mathrm{a}}}{\partial_{\upvarphi \mathrm{a}}}\right]=\left[\frac{v_{\ upvartheta \mathrm{b}}}{\mathrm{rb}}\frac{\partial {v}_{\mathrm{rb}}}{\mathrm{\partial \upvartheta b}}+\frac{v_{\ upvarphi \mathrm{b}}}{\mathrm{rb}\ \mathrm{sin}\upvartheta \mathrm{b}}\frac{\partial {v}_{\mathrm{rb}}}{\mathrm{\ gedeeltelijk \ upvarphi b}}-\frac{v_{\upvartheta \mathrm{b}}^2}{\mathrm{rb}}-\frac{v_{\upvarphi \mathrm{b}}^2}{\mathrm{rb }}+\frac{1}{\rho}\frac{\partial p}{\mathrm{\partial rb}}\right]/\left[\frac{1}{{\mathrm{rb}}^2 }\frac{\partial}{\mathrm{\partial rb}}\left({\mathrm{rb}}^2\frac{\partial {v}_{\mathrm{rb}}}{\mathrm{\ gedeeltelijk rb}}\right)+\frac{1}{{\mathrm{rb}}^2\mathrm{sin}\upvartheta \mathrm{b}}\frac{\partial}{\mathrm{\partial \upvartheta b}}\left(\mathrm{sin}\upvartheta \mathrm{b}\frac{\partial {v}_{\mathrm{rb}}}{\mathrm{\partial \upvartheta b}}\right)+ \frac{1}{{\mathrm{rb}}^2{\sin}^2\upvartheta \mathrm{b}}\frac{\partial^2{v}_{\mathrm{rb}}}{\ gedeeltelijk {\upvarphi \mathrm{b}}^2}-\frac{2{v}_{\mathrm{rb}}}{{\mathrm{rb}}^2}-\frac{2}{{\ mathrm{rb}}^2\sin \upvartheta \mathrm{b}}\frac{\partial \left({v}_{\upvartheta \mathrm{b}}\mathrm{sin}\upvartheta \mathrm{b} \right)}{\mathrm{\partial \upvartheta b}}-\frac{2}{{\mathrm{rb}}^2\mathrm{sin}\upvartheta \mathrm{b}}\frac{\partial { v}_{\upvarphi \mathrm{b}}}{\partial _{\upvarphi \mathrm{b}}}\right] $$ (11)Vergelijking 11 kan als volgt worden weergegeven:
$$ \mathrm{d}\upnu =\mathrm{rn}\left[{v}_{\upvartheta \mathrm{n}}\frac{\partial {v}_{\mathrm{rn}}}{\ mathrm{\partial \upvartheta n}}+\frac{v_{\upvarphi \mathrm{n}}}{\ \mathrm{sin}\upvartheta \mathrm{n}}\frac{\partial {v}_{\ mathrm{rn}}}{\mathrm{\partial \upvarphi n}}-{v}_{\upvartheta \mathrm{n}}^2-{v}_{\upvarphi \mathrm{n}}^2+ \frac{\mathrm{rn}}{\rho}\frac{\partial p}{\mathrm{\partial rn}}\right]/\left[\frac{\partial}{\mathrm{\partial rn} }\left({\mathrm{rn}}^2\frac{\partial {v}_{\mathrm{rn}}}{\mathrm{\partial rn}}\right)+\frac{1}{\ mathrm{sin}\upvartheta \mathrm{n}}\frac{\partial }{\mathrm{\partial \upvartheta n}}\left(\mathrm{sin}\upvartheta \mathrm{n}\frac{\partial { v}_{\mathrm{rn}}}{\mathrm{\partial \upvartheta n}}\right)+\frac{1}{\sin^2\upvartheta \mathrm{n}}\frac{\partial^ 2{v}_{\mathrm{rn}}}{\partial {\upvarphi \mathrm{n}}^2}-2{v}_{\mathrm{rn}}-\frac{2}{\sin \upvartheta \mathrm{n}}\frac{\partial \left({v}_{\upvartheta \mathrm{n}}\mathrm{sin}\upvartheta \mathrm{n}\right)}{\mathrm{\ par tial \upvartheta n}}-\frac{2}{\mathrm{sin}\upvartheta \mathrm{n}}\frac{\partial {v}_{\upvarphi \mathrm{n}}}{\partial_{\ upvarphi \mathrm{n}}}\right]=\mathrm{ra}\left[{v}_{\upvartheta \mathrm{a}}\frac{\partial {v}_{\mathrm{ra}}} {\mathrm{\partial \upvartheta a}}+\frac{v_{\upvarphi \mathrm{a}}}{\ \mathrm{sin}\upvartheta \mathrm{a}}\frac{\partial {v}_ {\mathrm{ra}}}{\mathrm{\partial \upvarphi a}}-{v}_{\upvartheta \mathrm{a}}^2-{v}_{\upvarphi \mathrm{a}}^ 2+\frac{\mathrm{ra}}{\rho}\frac{\partial p}{\mathrm{\partial ra}}\right]/\left[\frac{\partial}{\mathrm{\partial ra}}\left({\mathrm{ra}}^2\frac{\partial {v}_{\mathrm{ra}}}{\mathrm{\partial ra}}\right)+\frac{1} {\mathrm{sin}\upvartheta \mathrm{a}}\frac{\partial }{\mathrm{\partial \upvartheta a}}\left(\mathrm{sin}\upvartheta \mathrm{a}\frac{\ gedeeltelijk {v}_{\mathrm{ra}}}{\mathrm{\partial \upvartheta a}}\right)+\frac{1}{\sin^2\upvartheta \mathrm{a}}\frac{\ gedeeltelijk^2{v}_{\mathrm{ra}}}{\partial {\upvarphi \mathrm{a}}^2}-2{v}_{\mathrm{ra}}-\frac{2}{ \zonde \upvartheta \mathrm{a}}\frac{\partial \left({v}_{\upvartheta \mathrm{a}}\mathrm{sin}\upvartheta \mathrm{a}\right)}{\mathrm{\ gedeeltelijk \upvartheta a}}-\frac{2}{\mathrm{sin}\upvartheta \mathrm{a}}\frac{\partial {v}_{\upvarphi \mathrm{a}}}{\partial_{\ upvarphi \mathrm{a}}}\right]=\mathrm{rb}\left[{v}_{\upvartheta \mathrm{b}}\frac{\partial {v}_{\mathrm{rb}}} {\mathrm{\partial \upvartheta b}}+\frac{v_{\upvarphi \mathrm{b}}}{\ \mathrm{sin}\upvartheta \mathrm{b}}\frac{\partial {v}_ {\mathrm{rb}}}{\mathrm{\partial \upvarphi b}}-{v}_{\upvartheta \mathrm{b}}^2-{v}_{\upvarphi \mathrm{b}}^ 2+\frac{\mathrm{rb}}{\rho}\frac{\partial p}{\mathrm{\partial rb}}\right]/\left[\frac{\partial}{\mathrm{\partial rb}}\left({\mathrm{rb}}^2\frac{\partial {v}_{\mathrm{rb}}}{\mathrm{\partial rb}}\right)+\frac{1} {\mathrm{sin}\upvartheta \mathrm{b}}\frac{\partial }{\mathrm{\partial \upvartheta b}}\left(\mathrm{sin}\upvartheta \mathrm{b}\frac{\ gedeeltelijk {v}_{\mathrm{rb}}}{\mathrm{\partial \upvartheta b}}\right)+\frac{1}{\si n^2\upvartheta \mathrm{b}}\frac{\partial^2{v}_{\mathrm{rb}}}{\partial {\upvarphi \mathrm{b}}^2}-2{v} _{\mathrm{rb}}-\frac{2}{\sin \upvartheta \mathrm{b}}\frac{\partial \left({v}_{\upvartheta \mathrm{b}}\mathrm{sin }\upvartheta \mathrm{b}\right)}{\mathrm{\partial \upvartheta b}}-\frac{2}{\mathrm{sin}\upvartheta \mathrm{b}}\frac{\partial {v }_{\upvarphi \mathrm{b}}}{\partial_{\upvarphi \mathrm{b}}}\right] $$ (12)Er is een directe relatie tussen de stralen van de nanodeeltjes en de lymfeviscositeit door kanker. Als lymfe te statisch en stroperig wordt, kan het zijn functie niet goed uitoefenen, namelijk de circulatie en het opruimen van gifstoffen en het helpen bestrijden van kanker. Als de nanodeeltjes kleiner zijn, zijn de lymfatische kankercellen gemakkelijk te doden. Om het transport van de totale hoeveelheid van de samengestelde nanodeeltjes te beschrijven, gebruiken we de continuïteitsvergelijking en nemen we de drie nanodeeltjes van vaste stoffen A, B en N als volgt aan:
$$ \frac{1}{{\mathrm{ra}}^2}\frac{\partial }{\mathrm{\partial ra}}\left({\mathrm{ra}}^2{v}_{ \mathrm{ra}}\right)+\frac{1}{\mathrm{ra}\ \mathrm{sin}\upvartheta \mathrm{a}}\frac{\partial}{\mathrm{\partial \upvartheta a }}\left(\sin {\upvartheta \mathrm{v}}_{\upvartheta \mathrm{a}}\right)+\frac{1}{\mathrm{ra}\ \mathrm{sin}\upvartheta \ mathrm{a}}\frac{\partial {v}_{\upvarphi \mathrm{a}}}{\mathrm{\partial \upvarphi a}}+\frac{1}{{\mathrm{rb}}^ 2}\frac{\partial}{\mathrm{\partial rb}}\left({\mathrm{rb}}^2{v}_{\mathrm{rb}}\right)+\frac{1}{ \mathrm{rb}\ \mathrm{sin}\upvartheta \mathrm{b}}\frac{\partial }{\mathrm{\partial \upvartheta b}}\left(\sin {\upvartheta v}_{\upvartheta \mathrm{b}}\right)+\frac{1}{\mathrm{rb}\ \mathrm{sin}\upvartheta \mathrm{b}}\frac{\partial {v}_{\upvarphi \mathrm{ b}}}{\mathrm{\partial \upvarphi b}}+\frac{1}{{\mathrm{rn}}^2}\frac{\partial}{\mathrm{\partial rn}}\left( {\mathrm{rn}}^2{v}_{\mathrm{rn}}\right)+\frac{1}{\mathrm{rn}\ \mathrm{sin}\upvartheta \mathrm{n}}\ frac{\parti al }{\mathrm{\partial \upvartheta n}}\left(\sin {\upvartheta v}_{\upvartheta \mathrm{n}}\right)+\frac{1}{\mathrm{rn}\ \ mathrm{sin}\upvartheta \mathrm{n}}\frac{\partial {v}_{\upvarphi \mathrm{n}}}{\mathrm{\partial \upvarphi n}}=0 $$ (13)De dynamische vloeistofviscositeit kan worden bepaald met de volgende vergelijking [23]:
$$ \mathrm{Vs}=\frac{2}{9}\frac{r^2g\ \left(\uprho \mathrm{p}-\uprho \mathrm{f}\right)}{\mathrm{dv }} $$ (14)where Vs is the particles’ settling velocity (m/s), r is the Stokes radius of the particle (m), g is the gravitational acceleration (m/s 2 ), ρp is the density of the particles (kg/m 3 ), ρf is the density of the fluid (kg/m 3 ), and dv is the (dynamic) fluid viscosity (Pa·s). The lymph fluid is slightly heavier than water (lymph density = 1019 kg/m 3 , water density = 998.28 kg/m 3 at 20 °C). As a reference value, we consider the dynamic viscosity of the water to be 1.002 × 10 –3 kg m –1 s –1 ).
Dynamic viscosity is the measurement of the fluid’s internal resistance to flow, while kinematic viscosity refers to the ratio of dynamic viscosity to density. The effect of all the nanoparticles on the fluid viscosity is represented as follows:
$$ \mathrm{dv}=\frac{2\mathrm{g}}{9}\left[\frac{{\mathrm{ra}}^2\left(\uprho \mathrm{a}-\uprho \mathrm{f}\right)}{\mathrm{vsa}}+\frac{{\mathrm{rb}}^2\left(\uprho \mathrm{b}-\uprho \mathrm{f}\right)}{\mathrm{vsb}}+\frac{{\mathrm{rn}}^2\left(\uprho \mathrm{n}-\uprho \mathrm{f}\right)}{\mathrm{vn}}\right] $$ (15)By comparing Eq. 12 and Eq. 15, the following equation could be emerged:
$$ \left|\frac{2\mathrm{g}}{9}\left[\frac{{\mathrm{ra}}^2\left(\uprho \mathrm{a}-\uprho \mathrm{f}\right)}{\mathrm{vsa}}+\frac{{\mathrm{rb}}^2\left(\uprho \mathrm{b}-\uprho \mathrm{f}\right)}{\mathrm{vsb}}+\frac{{\mathrm{rn}}^2\left(\uprho \mathrm{n}-\uprho \mathrm{f}\right)}{\mathrm{vn}}\right]\right|=\mathrm{rn}\left[{v}_{\upvartheta \mathrm{n}}\frac{\partial {v}_{\mathrm{rn}}}{\mathrm{\partial \upvartheta n}}+\frac{v_{\upvarphi \mathrm{n}}}{\ \mathrm{sin}\upvartheta \mathrm{n}}\frac{\partial {v}_{\mathrm{rn}}}{\mathrm{\partial \upvarphi n}}-{v}_{\upvartheta \mathrm{n}}^2-{v}_{\upvarphi \mathrm{n}}^2+\frac{\mathrm{rn}}{\uprho \mathrm{f}}\frac{\mathrm{\partial p}}{\mathrm{\partial rn}}\right]/\left[\frac{\partial }{\mathrm{\partial rn}}\left({\mathrm{rn}}^2\frac{\partial {v}_{\mathrm{rn}}}{\mathrm{\partial rn}}\right)+\frac{1}{\mathrm{sin}\upvartheta \mathrm{n}}\frac{\partial }{\mathrm{\partial \upvartheta n}}\left(\mathrm{sin}\upvartheta \mathrm{n}\frac{\partial {v}_{\mathrm{rn}}}{\mathrm{\partial \upvartheta n}}\right)+\frac{1}{\sin^2\upvartheta \mathrm{n}}\frac{\partial^2{v}_{\mathrm{rn}}}{\partial {\upvarphi \mathrm{n}}^2}-2{v}_{\mathrm{rn}}-\frac{2}{\sin \upvartheta \mathrm{n}}\frac{\partial \left({v}_{\upvartheta \mathrm{n}}\mathrm{sin}\upvartheta \mathrm{n}\right)}{\mathrm{\partial \upvartheta n}}-\frac{2}{\mathrm{sin}\upvartheta \mathrm{n}}\frac{\partial {v}_{\upvarphi \mathrm{n}}}{\partial_{\upvarphi \mathrm{n}}}\right]=\mathrm{ra}\left[{v}_{\upvartheta \mathrm{a}}\frac{\partial {v}_{\mathrm{ra}}}{\mathrm{\partial \upvartheta a}}+\frac{v_{\upvarphi \mathrm{a}}}{\ \mathrm{sin}\upvartheta \mathrm{a}}\frac{\partial {v}_{\mathrm{ra}}}{\mathrm{\partial \upvarphi a}}-{v}_{\upvartheta \mathrm{a}}^2-{v}_{\upvarphi \mathrm{a}}^2+\frac{\mathrm{ra}}{\uprho \mathrm{f}}\frac{\partial p}{\mathrm{\partial ra}}\right]/\left[\frac{\partial }{\mathrm{\partial ra}}\left({\mathrm{ra}}^2\frac{\partial {v}_{\mathrm{ra}}}{\mathrm{\partial ra}}\right)+\frac{1}{\mathrm{sin}\upvartheta \mathrm{a}}\frac{\partial }{\mathrm{\partial \upvartheta a}}\left(\mathrm{sin}\upvartheta \mathrm{a}\frac{\partial {v}_{\mathrm{ra}}}{\mathrm{\partial \upvartheta a}}\right)+\frac{1}{\sin^2\upvartheta \mathrm{a}}\frac{\partial^2{v}_{\mathrm{ra}}}{\partial {\upvarphi \mathrm{a}}^2}-2{v}_{\mathrm{ra}}-\frac{2}{\sin \upvartheta \mathrm{a}}\frac{\partial \left({v}_{\upvartheta \mathrm{a}}\mathrm{sin}\upvartheta \mathrm{a}\right)}{\mathrm{\partial \upvartheta a}}-\frac{2}{\mathrm{sin}\upvartheta \mathrm{a}}\frac{\partial {v}_{\upvarphi \mathrm{a}}}{\partial_{\upvarphi \mathrm{a}}}\right]=\mathrm{rb}\left[{v}_{\upvartheta \mathrm{b}}\frac{\partial {v}_{\mathrm{rb}}}{\mathrm{\partial \upvartheta b}}+\frac{v_{\upvarphi \mathrm{b}}}{\ \mathrm{sin}\upvartheta \mathrm{b}}\frac{\partial {v}_{\mathrm{rb}}}{\mathrm{\partial \upvarphi b}}-{v}_{\upvartheta \mathrm{b}}^2-{v}_{\upvarphi \mathrm{b}}^2+\frac{\mathrm{rb}}{\uprho \mathrm{f}}\frac{\partial p}{\mathrm{\partial rb}}\right]/\left[\frac{\partial }{\mathrm{\partial rb}}\left({\mathrm{rb}}^2\frac{\partial {v}_{\mathrm{rb}}}{\mathrm{\partial rb}}\right)+\frac{1}{\mathrm{sin}\upvartheta \mathrm{b}}\frac{\partial }{\mathrm{\partial \upvartheta b}}\left(\mathrm{sin}\upvartheta \mathrm{b}\frac{\partial {v}_{\mathrm{rb}}}{\mathrm{\partial \upvartheta b}}\right)+\frac{1}{\sin^2\upvartheta \mathrm{b}}\frac{\partial^2{v}_{\mathrm{rb}}}{\partial {\upvarphi \mathrm{b}}^2}-2{v}_{\mathrm{rb}}-\frac{2}{\sin \upvartheta \mathrm{b}}\frac{\partial \left({v}_{\upvartheta \mathrm{b}}\mathrm{sin}\upvartheta \mathrm{b}\right)}{\mathrm{\partial \upvartheta b}}-\frac{2}{\mathrm{sin}\upvartheta \mathrm{b}}\frac{\partial {v}_{\upvarphi \mathrm{b}}}{\partial_{\upvarphi \mathrm{b}}}\right] $$ (16)Equation 16 depicts the relationship between the density of the lymph fluid, the density of the nanoparticles of the compound drug system, and the radius of the nanoparticles. There is a positive correlation between the density of the lymph fluid and the density of the nanoparticles. The smaller the density and radius of nanoparticles, the lesser the density of the fluid will be. As established earlier, the decrease in the density of the lymph fluid leads to its inability to reproduce and reduce the ferocity of the disease. It can, therefore, be concluded that the tumor can be cured by minimizing the size of nanoparticles. These particles can reach in the range of up to 0.1 nm (i.e., Angstrom or picometer range). The particles of this size can act as the nucleus of drug delivery in this drug system. Equation 16 shows that radii of the nanoparticles in the proposed drug system are related to the effectiveness of the delivery system. Much lower sized nanoparticles can reduce the density of lymph fluid and the spread of the disease.
Nanoparticles with Nanoantennas
This study used the nanoparticle described in an earlier report [22] as an emissary with a nano-microcontroller. In the system, the proposed transmission distance is very small and compatible with the composition of nanoparticles. Thus, the middle gap can be neglected in mid-distance and is symbolized by C d . Further, R een en X een are the real part and the imaginary part of the anaerobic impedance. After neglecting the load of the intercellular space between the nanoparticles and the nano-microcontroller, R een en X een can be calculated as follows [22]:
$$ {R}_a=\frac{r_{a0}}{1+{C}_d{w}_a\left(2{x}_{a0}+{C}_d\left({r_{a0}}^2+{x_{a0}}^2\right){w}_a\right)} $$ (17) $$ {X}_a=\frac{x_0-{C}_d\left({r_{a0}}^2+{x_{a0}}^2\right){w}_a}{1+{C}_d{w}_a\left(2{x}_{a0}+{C}_d\left({r_{a0}}^2+{x_{a0}}^2\right){w}_a\right)} $$ (18)Thus, the load resistance value of nanotubes can be predicted as in the following equation:
$$ {r}_l=\frac{g^2R}{g^2-2 gSX{\varepsilon}_L\omega +{S}^2\left({R}^2+{X}^2\right){\varepsilon_L}^2{\omega}^2} $$ (19)waar ε L is the permittivity of the loading material, g is the size of the gap, and S is the effective cross-section area of the gap. In order to simplify the equation, the value of g 2 can be neglected as it is too low and the final equation can be rewritten as follows:
$$ {r}_l=\frac{g^2R}{-2 gSX{\varepsilon}_L\omega +{S}^2\left({R}^2+{X}^2\right){\varepsilon_L}^2{\omega}^2} $$ (20)Then,
$$ {r}_l=\frac{g^2R}{S{\varepsilon}_L\omega \left(-2 gX+S\left({R}^2+{X}^2\right){\varepsilon}_L\omega \right)} $$ (21)The optical nano-photo concept can be used as an effective tool for interpreting and predicting these effects to design and improve nanoscale parameters and increase the nano-sensitivity to serve better as a single molecular sensor. Nanoantenna may provide optimal performance in terms of sensitivity, efficiency, and bandwidth in the process. The next section presents the concept of searching the cancerous lymph nodes using compressive binary search algorithm.
Searching for the Target Lymphatic Nodes Using Compressive Binary Search
In order for nanoparticles to reach the cancerous cells in a fast and efficient manner, we applied compressive binary search by the nano-microcontroller. The guided nanoparticles follow a specific path to quickly reach the target. This movement is based on the information obtained from the “exploratory nanoparticles.” Assume that the target lymph node, Tf, has exactly one nonzero entry, where the location of the lymph node is unknown. The algorithm divides mt measurements into a total of St stages, where St refers to the stages of the lymph nodes. The measurements are more than one for all the stages of the lymph nodes, which is necessary for the algorithm to be executed until completion. Based on this measurement, the algorithm decides between going left or right, until the nanoparticles reach the target, the cancerous lymph node.
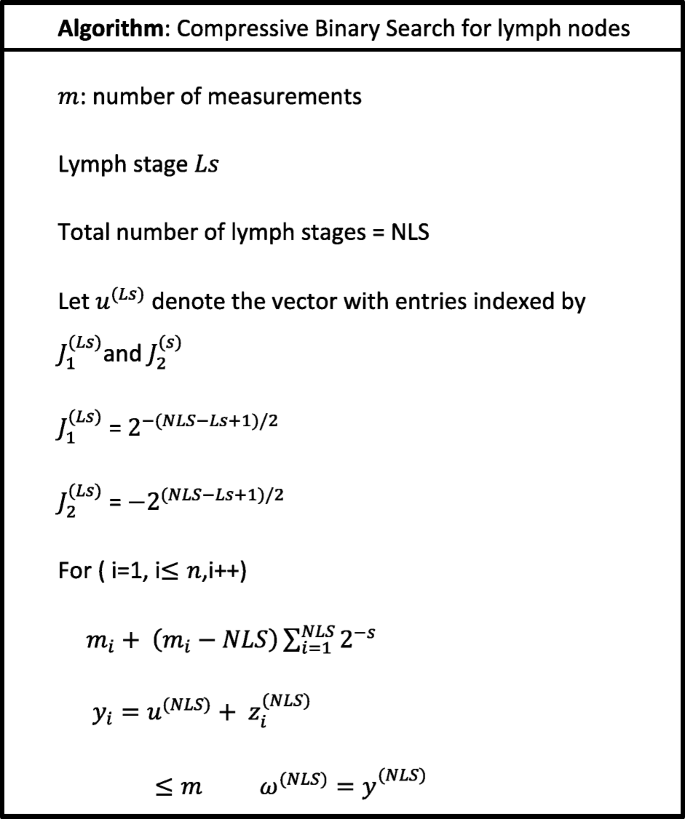
Resultaten en discussie
In order to analyze the proposed design, the nanoparticles were applied to the following five types of materials:silicone, lithium, lung, helium, and hydrogen. The materials were chosen because of their low density. The lung nanoparticles were samples from nano-sized lung nodules. They appear encircling with white shadows in a chest X-ray or computerized tomography scan taken from the lung of the person and required to be undamaged. The proposed idea is based on the analytical model, which indicates that the smaller the density of nanoparticles, the smaller the dynamic viscosity will be. This will result in a decrease in fluid viscosity. It is shown that the types of materials and the density of each particle will affect settling velocity of nanoparticles at entry into the lymphatic fluid and the density of the lymphatic fluid. We considered the following parameters:acceleration of gravity (g ) = 9.80665, particle diameter (d ) = 10 A, initial density of lymph fluid (ρf) = 998.28, and dynamic viscosity = 0.0010 kg m –1 s –1 [24]. These parameters were selected by the assumption that the viscosity of the lymphatic fluid is very similar to the viscosity of the water and the very small difference does not affect the results of the model. Figure 4 illustrates the density of nanoparticles for five selected materials for application in the proposed analytical model. Figure 5 shows the settling velocity for each particle. Figure 6 shows the effect of the settling velocity of nanoparticles on altering the lymphocyte density of cancer cells. The results shown in Fig. 6 show that the settling velocity of the particles carries a negative value. This indicates that the nanoparticle after entering in the lymphatic fluid rapidly moves in the opposite direction toward their entry into the lymphatic fluid. In general, any object that moves in the negative direction has a negative velocity. This movement of the particle leads to reduced viscosity of the lymphatic fluid.
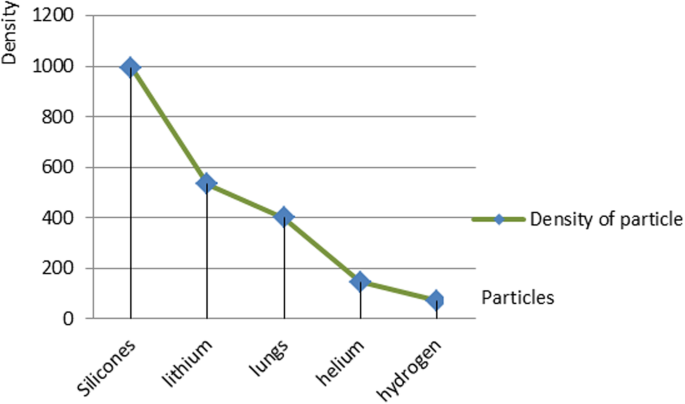
Density of nanoparticles for the five selected materials
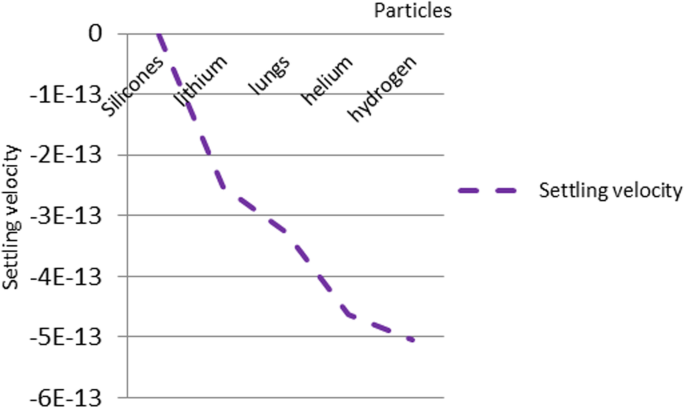
The settling velocity data for each particle
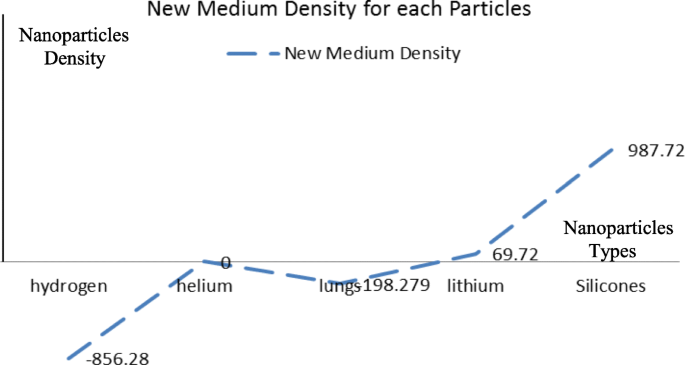
The effect of the settling velocity of nanoparticles on changing the lymphocyte density of cancer cells
Silicone nanoparticles showed the settling velocity of approximately − 2.87 × 10–15 m/s. This resulted in a decrease in viscosity of the lymphatic fluid to 987.72 kg/m 3 for the initial density 998.28 kg/m 3 . The density is continuously reduced to a point where hydrogen produces extremely spectacular results, i.e., the complete collapse of lymphatic fluid resistance. The density of the lymphatic fluid − 856.28 kg/m 3 with the negative sign indicated that there was no resistance from the lymphatic fluid to the flow of the nanoparticles, resulting in the complete collapse of the liquid fluid. Both the hydrogen and helium particles have a significant impact on the liquid viscosity due to the low density of the particles. Hence, it is important to use a drug system consisting of a group of nanoparticles for low-density materials. Figure 7 shows the relationship between the diameters of lung nanoparticles and the number of nanoparticles in one group. The figure shows that the higher the diameter of nanoparticles, the fewer their number in a group. This is clearly shown at the highest value of the nanoparticle diameter of 1000 nm, where the number of molecules in a group is 20 molecules. Figure 8 shows the relationship between the diameters of lithium nanoparticles and the number of nanoparticles in one group. This figure demonstrates the inverse relationship between the radius of nanoparticles and the number of molecules in a group where lithium particle diameters are significantly lower than the lung nanoparticles, where the number of nanoparticles in Fig. 7 is relatively low compared to the lithium particles as shown in Fig. 8. And the multicolor balls in both figures refer to different ranges of nanoparticle radii for each group, where each group contains a number of nanoparticles with different sizes. The best results can be obtained when hydrogen and helium particles are increased from other substances. A mixture of different materials should be used so that the properties of these substances can be used in the treatment process as well as to reduce viscosity. Figure 9 illustrates the different sets of materials proposed to have the mean highest density of both hydrogen and helium materials. Figure 10 shows the average mass of a nanoparticle in a group. It can be seen that the mass of both hydrogen and helium is the highest compared to the mass of particles of other substances. Figure 11 illustrates the relationship between the diameters of the nanoparticles and the width of its group or class. It is important to note that these results will open up a new area to reduce the resistance of the lymphatic fluid in tumors. This can be achieved using hydrogen nanoparticles of a size in the range of Angstrom. In addition to hydrogen nanoparticles, there may also exist a number of other substances in the same size. Figure 12 illustrates the standard deviation of a number of coefficients for both lung and lithium nanoparticles. These coefficients are limited to fractions of nanoparticles in a single group as well as their number in addition to the diameters of these nanoparticles. It is clear that the group fractions have the less value of the standard deviation. Hence, most of the fractions in the computational processes are around the mean of these values. Figure 13 shows the standard deviation of the mass for particles of silicones, lithium, lungs, helium, and hydrogen in one group. It is clear that the particles of the lung have the largest standard deviation and the lithium has the minimum value.
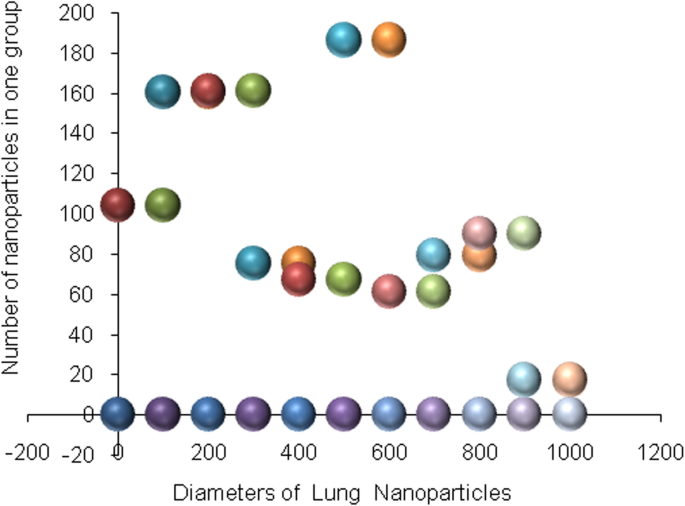
Group of nanoparticles in the lung cells and their number in one of the proposed groups
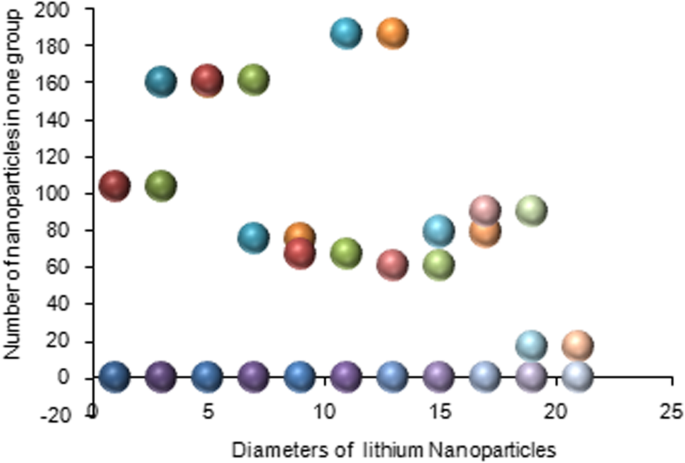
Group of nanoparticles in the lithium cells and their number in one of the proposed groups
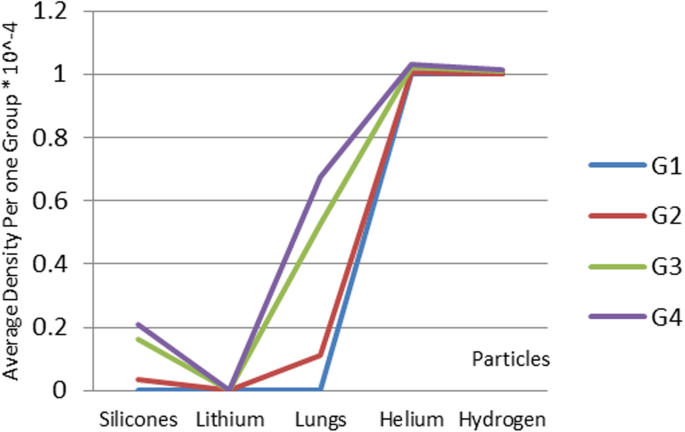
Different sets of materials proposed to have the mean highest density of both hydrogen and helium materials
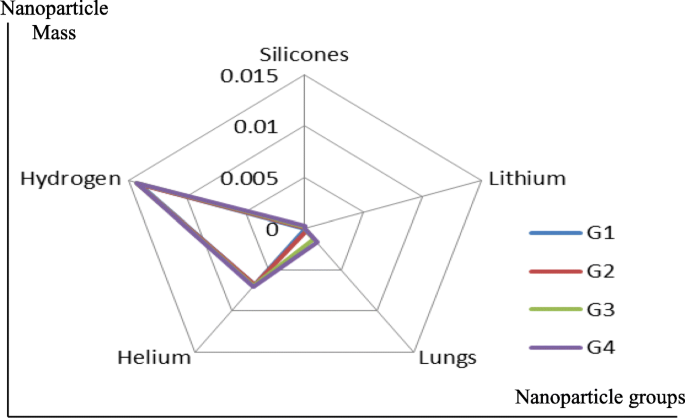
Average mass of a nanoparticle in a group
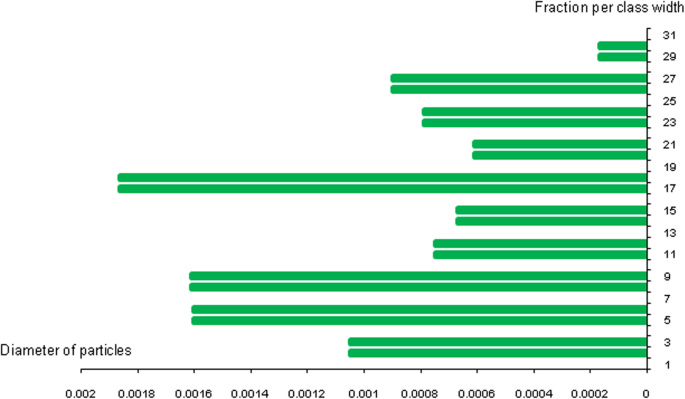
Diameters of the nanoparticles related to the group width
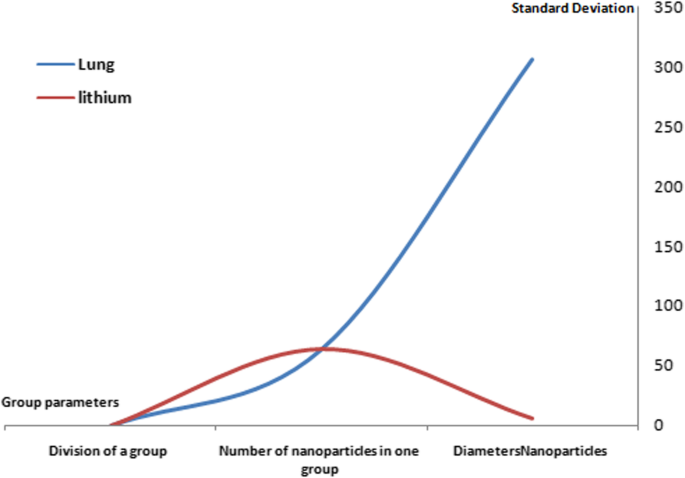
The standard deviation of lung and lithium nanoparticles coefficients
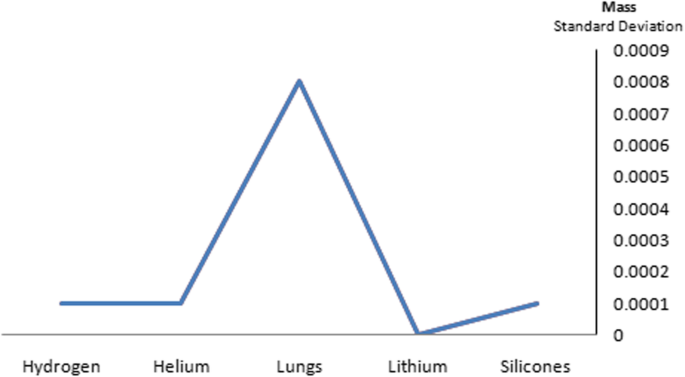
The standard deviation of the mass for particles of silicones, lithium, lungs, helium, and hydrogen in one group
Methoden
The aim of this study was to establish a nano-drug delivery system capable of delivering the drugs effectively to the cancer cells. The following methodology was used to deliver nanoparticles:
- i)
Low-density nanoparticles
This study proposed the theoretical approach of nanoparticles as a low-density drug. This depends on the density and the settling velocity of the nanoparticles, as these nanoparticles can overcome the resistance of the lymphatic fluid.
- ii)
Preparation of anaerobic nanoparticles
This study uses the idea of nanoparticles possessing an antenna through which a connection can be made between nanoparticles and nano-controller. The transmission distance was assumed to be too small to match the composition of nanoparticles and also to fit the actual distance between them.
- iii)
Nano-controller design
Its function is to deliver the nanoparticle drug to cancer cells. Its role is to send signals to the nanoparticles and coordinate their actions and direct them to the lymphatic fluid of tumors.
- iv)
Searching for the target lymphatic nodes
The lymphatic nodes are searched using compressive binary search algorithm. This algorithm is characterized by high-speed search, which makes nanoparticles more accessible to infected cells than the conventional methods. The primary supervisor behind the performance of the nanoparticles is the nano-controller. It directs nanoparticles to the infected cells by following this algorithm to ensure that an appropriate number of molecules are in proportional density to the lymphatic fluid.
Conclusie
There have been various studies managing the treatment of malignant growth utilizing nanoparticles. The lymphatic liquid in tumors plays a substantial role in the obstruction of medication to the cancer cells. We developed an intelligent drug delivery system containing a consortium of nanoparticles. The proposed design demonstrates that small nanoparticles result in low density of the fluid. The results indicated that hydrogen particles are most efficient in reducing resistance toward lymphatic liquid owing to their smaller size. Furthermore, the design involves an anaerobic nano-controller that can determine the state and area of the particles. This technique conveys the medication to the infected cell more effectively.
Beschikbaarheid van gegevens en materialen
The datasets supporting the results of this article are included within the article.
Afkortingen
- LN:
-
Lipide nanodeeltjes
- NLC:
-
Nanostructured lipid carriers
- PN:
-
Polymeric nanoparticles
- SLN's:
-
Vaste lipide nanodeeltjes
Nanomaterialen
- Fail-safe ontwerp
- Nanovezels en filamenten voor verbeterde medicijnafgifte
- Biocompatibele FePO4-nanodeeltjes:medicijnafgifte, RNA-stabilisatie en functionele activiteit
- Omgevingsgevoelige metaal-organische raamwerken als medicijnafgiftesysteem voor tumortherapie
- Op cellen gebaseerde medicijnafgifte voor kankertoepassingen
- Zebravis:een veelbelovend real-time modelsysteem voor door nanotechnologie gemedieerde neurospecifieke medicijnafgifte
- 131I-getraceerde PLGA-lipide nanodeeltjes als dragers van medicijnafgifte voor de gerichte chemotherapiebehandeling van melanoom
- De studie van een nieuw, door nanodeeltjes versterkt, wormachtig micellair systeem
- Nanotechnologie:van in vivo beeldvormingssysteem tot gecontroleerde medicijnafgifte
- Endotheelcellen targeten met multifunctionele GaN/Fe-nanodeeltjes
- Silica-nanodeeltjes voor intracellulaire eiwitafgifte:een nieuwe synthesebenadering met behulp van groene fluorescerende eiwitten