Kleine zeldzame-aarde-fluoride-nanodeeltjes activeren tumorcelgroei via elektrische polaire interacties
Abstract
Gelokaliseerde extracellulaire interacties tussen nanodeeltjes en transmembraansignaalreceptoren kunnen de groei van kankercellen mogelijk activeren. Hierin, kleine LaF3 en PrF3 nanodeeltjes in DMEM+FBS-suspensies stimuleerden tumorcelgroei in drie verschillende menselijke cellijnen (A549, SW837 en MCF7). Grootteverdeling van nanodeeltjes, activering van AKT- en ERK-signaleringsroutes en levensvatbaarheidstesten wezen op mechanische stimulatie van ligandadhesiebindingsplaatsen van integrines en EGFR via een synergetische werking van een ensemble van kleine nanodeeltjes (<-10 nm). Hoewel nanodeeltjes van kleine afmetingen goed geassocieerd kunnen worden met de activering van EGFR, blijft de wisselwerking tussen integrine en nanodeeltjes een veelzijdig probleem. Een theoretisch motief laat zien dat, binnen de vereiste pN-krachtschaal, elke ligandadhesiebindingsplaats kan worden geactiveerd door een klein diëlektrisch nanodeeltje via elektrische dipoolinteractie. De grootte van het actieve nanodeeltje bleef gespecificeerd door de hoeveelheid oppervlakteladingen op de ligandadhesiebindingsplaats en het nanodeeltje, en ook door de scheidingsafstand ertussen. De polaire component van de elektrische dipoolkracht bleef omgekeerd evenredig met de tweede macht van de grootte van nanodeeltjes, wat aantoont dat alleen kleine diëlektrische nanodeeltjes de groei van kankercellen kunnen stimuleren via elektrische dipoolinteracties. Het werk draagt bij aan het herkennen van verschillende cytoskelet-stress-modi van kankercellen.
Achtergrond
Tumorogenese is een multidimensionaal probleem waarbij genomische veranderingen betrokken zijn. Het wordt ook geactiveerd door cel-extracellulaire matrix (ECM) interacties tussen scaffolds en cytoskeletstructuren [1,2,3,4] uitgedrukt via stress van mechanosensoren, vergelijkbaar met integrines, van meerdelige cellulaire krachten die in staat zijn de genomische programmering te veranderen [5]. De interacties van de tumormicro-omgeving met ECM-steigers activeren gewoonlijk de focale adhesie-eiwitten van het celmembraan en transmembraansignaalreceptoren (TSR), epidermale groeifactorreceptoren (EGFR), vasculaire endotheliale groeifactor (VEGFR) of zenuwgroeifactorreceptoren (NGFR). De mechanosensoren reguleren de groei van tumorcellen via signaaltransacties tussen het extracellulaire actieve domein van cellen [6,7,8,9] en de intracellulaire F-actinefilamenten, door een lawine van fosforyleringsreacties op gang te brengen.
Eiwitconformatieveranderingen en excitatie van TSR-routes vereisen dat de activerende kracht in het pN-krachtbereik ligt, en zeker onder de nN-meter [10]. Naast willekeurige mechanische belasting en actieve chemische affiniteitssterkte, kan de bindingsefficiëntie (sterkte van binding) tussen nanodeeltjes (NP's) en de eiwitten van het celmembraan worden gemoduleerd via korte of lange afstand elektrische polaire of andere soorten dispersieve interacties. Op het beperkte oppervlak van NP's kan slechts een bepaald aantal eiwitten lang genoeg worden vastgehecht om biologisch actief te zijn [11], en in de ruimte beperkte lokale interacties met het biologische milieu werden erkend als verantwoordelijk voor een reeks uiteenlopende celfunctionaliteiten trajecten [12]. Bijgevolg signaleren de signaaltransactieroutes van eiwit-NP's-interactie veiligheidsproblemen voor NP's [11, 13].
Aangezien een gunstige of ongunstige respons in cellen van NP's typespecifiek is [11], moet het verband tussen NP's en biologische kenmerken van geval tot geval worden vastgesteld [14, 15].
Tegenstrijdige resultaten van tumorcellen die zijn blootgesteld aan NP's, voor ablatieve of tumorgroei-efficiëntie of variabele toxiciteitsniveaus van NP's [16, 17], brengen ook de veiligheidsproblemen naar voren. Ondanks de vooruitgang is er vandaag de dag echter een gebrek aan kennis over de specifieke routes waarmee NP's interageren met eukaryote cellen, waardoor de identificatie van een universele NP-therapeutische benadering onmogelijk is. Omdat NP's van verschillende grootte en diverse oppervlaktechemie gewoonlijk cellulaire reacties afleiden, waaronder NP's-membraanreceptorbinding en TSR's-activiteit, is de toxiciteit van NP's gerelateerd aan de morfologie van oppervlakteactieve stoffen, de elektrische laadtoestand, de concentratie en samenstelling van eiwitten en nanomaterialen in ECM [18,19,20,21] en ten slotte de sterkte van de moleculaire binding tussen NP's en de celfenotypes [22].
Eerdere studies van melanoom en baarmoederhalscarcinomen blootgesteld aan silica, gouden NP's en koolstofnanobuisjes erkenden dat de grootte van NP's tumorcelgroei selectief activeert [23,24,25,26,27]. De correlatie tussen TSR-signalen in menselijke SK-BR-3 kankercellijn en de grootte van gemodificeerde gouden en zilveren NP's toonde aan dat hoewel 2-100 nm grootte NP's de signaaltransductie hervormden, er een enorm verschil in apoptotische activiteit werd bereikt wanneer cellen interageerden met 40 NP's met een grootte van 50 nm [26]. Onlangs werd ook gesuggereerd dat het veranderen van de grootte van gouden NP's van 5 naar 40 nm, de groeisnelheden van A549- en 95D-kankercellijnen mogelijk waren afgestemd. Specifiek remden NP's met een grootte van 5 nm elke proliferatie van beide celtypen, terwijl NP's van ongeveer 10 nm geen effect hadden op de celgroei [27]. Evenzo werden A549- en THP-1-cellen blootgesteld aan SiO2 NP's vertoonden grootteafhankelijke cytotoxiciteit en NP's van 15 nm waren ook gecorreleerd met hoge cytotoxiciteitsniveaus. Integendeel, NP's met een grootte van 60 nm vertoonden een lagere toxiciteit. Ten slotte verhoogden NP's met een grootte van 200 nm de groei van stamcellen door ERK1/2-activering, terwijl NP's met een grootte van 2-4 m verschillende signaaltransductieroutes konden activeren [28]. Kleine NP's conjugeren de EGFR en schakelen de eiwitkinase B (AKT) en extracellulaire signaal-gereguleerde kinase (ERK) signaaltransactieroutes in die de celgroei stimuleren.
Zeldzame-aarde nanodeeltjes (RE-NP's) kunnen ook interageren met specifieke domeinen zoals metaalion-afhankelijke adhesieplaatsen (MIDAS), aanpassing aan MIDAS (ADMIDAS), synergetische metaalionbindingsplaatsen (SyMBS) en ligandadhesiebindingsplaatsen (LABS) , gelegen in de αν β3 subeenheid of andere subeenheden van integrines [29, 30].
Evenzo brengen RE-NP's een extra mate van soepelheid met zich mee in de interactie van tumor-NP's [31,32,33,34]. Terwijl ceria-NP's (nanoceria) een beschermende werking vertoonden tegen cellulaire schade door verschillende radicalen [35], versterkten lage concentratieniveaus van gemodificeerde ceria-NP's de hepatoomcelproliferatie sterk door apoptose te verminderen via activering van AKT/ERK-signaleringsroutes [36]. Typisch is een ensemble van NP's die de cel omringen verantwoordelijk voor cytoskeletale stress en evenzeer opkomen voor chemische, nano-thermodynamische (Hill) [37], entropische of een elektrische dipoolinteractie tussen NP's en mechanosensoren. Tot nu toe blijft een omhulsel van begrip van interacties tussen NP's, TSR's en cellen echter vaag en onbeschikbaar.
In principe zou het sterke ionische karakter van RE-verbindingen de mechanosensoren van cellen moeten stimuleren via elektrische interacties. Omdat RE-ionen veel worden gebruikt in verschillende toepassingen, is het ook van vitaal belang om hun potentiële bijdrage aan de groei van tumorcellen te onderzoeken voor het impliceren van geschikte protocollen voor de bescherming van de volksgezondheid. Lanthaanfluoride (LaF3 ) en praseodymiumfluoride (PrF3 ) worden gebruikt in fluorescentielampen, stralingskleurenglazen, glasvezel, emailtoepassingen en elektroden. LaF3 wordt uitgewerkt in een specifiek type glas, fosforlampcoatings, waterbehandeling en katalysatoren. Het is ook een essentieel onderdeel van een commercieel fluorideglas (ZBLAN), dat gemengd met europiumfluoride wordt gebruikt voor optische communicatie en als kristalmembraan in ion-selectieve fluoride-elektroden met een goede doorlaatbaarheid in het infrarood. Evenzo, PrF3 wordt ook gebruikt in koolstofbooglampen voor de filmindustrie, studioverlichting en projectorlampen. Fluorideglazen gedoteerd met praseodymium worden ook gebruikt in single-mode glasvezelversterkers.
Daarbij toont dit werk aan dat RE-NP's van kleine omvang de potentie hadden om tumorcelgroei te stimuleren via elektrische dipoolinteracties.
Het artikel is onderverdeeld in drie secties. Eerst worden de grootteverdeling, de interacties en de geometrie van NP's geanalyseerd door toepassing van dynamische lichtverstrooiing (DLS), atoomkrachtmicroscopie (AFM), transmissie-elektronenmicroscopie (TEM), röntgendiffractie (XRD), tweedimensionaal snel Fourier-transformatie (2D-FFT) analyse en vacuüm ultraviolet spectroscopie (VUV 110-180 nm). Vervolgens de correlatie tussen het groeiniveau van drie verschillende menselijke kankercellijnen (A549, SW837 en MCF7) met de grootteverdeling en de concentratie van LaF3 en PrF3 NP wordt opgericht. Ten slotte, binnen een vereiste krachtlimiet van 1 pN voor het activeren van mechanosensoren en vervolgens voor tumorcelgroei, wordt de levensvatbaarheid van tumorcellen gepast binnen een theoretisch motief van elektrische dipoolinteractie tussen één RE-NP en één LABS. Het werk draagt bij aan de identificatie en classificatie van verschillende soorten cytoskeletale stress en interacties tussen NP's en mechanosensorische kankercellen.
Resultaten
Grootte en structuur van NP's
Ten eerste, DLS, AFM, TEM, XRD, FFT, VUV-spectroscopie en t teststatistieken werden toegepast om de grootteverdeling van RE-NP's in vloeibare suspensies te extraheren (figuren 1, 2, 3, 4, 5, 6 en 7). Vervolgens werden levensvatbaarheidstesten van cellen en Western blotting (Wb) -assays gebruikt om de activering van de specifieke mechanosensoren door RE-NP's te identificeren.
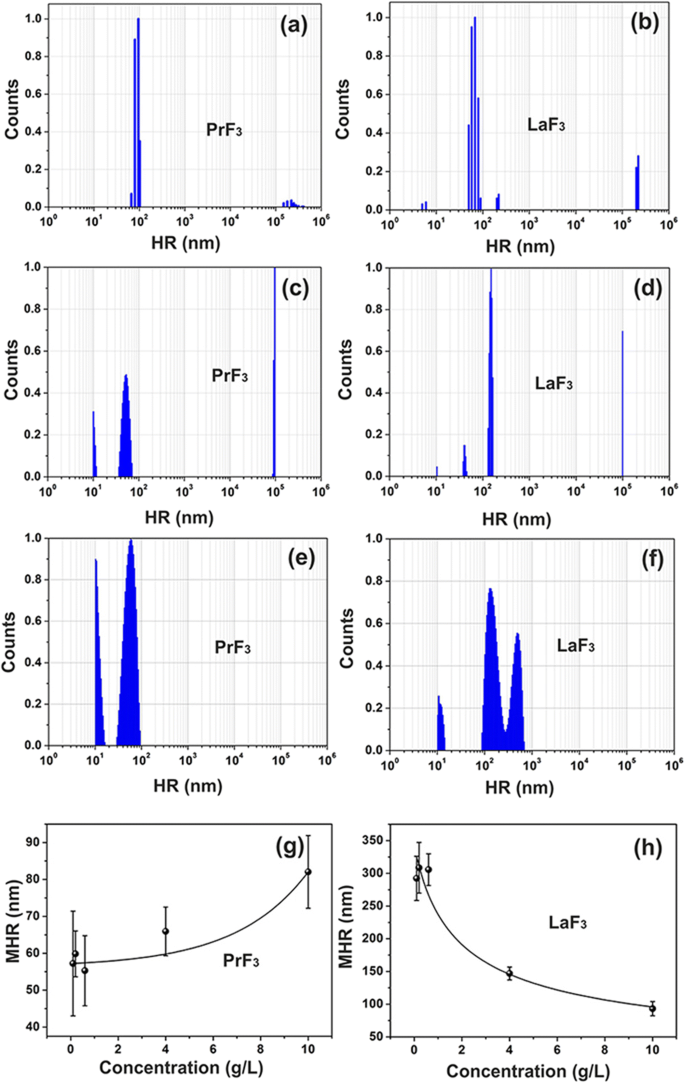
DLS-maatverdelingsspectra van RE-suspensies. a, b PrF3 en LaF3 NP's (5 g/L) in water. c, d PrF3 en LaF3 NP's (5 g/L) in DMEM+FBS. e, f PrF3 en LaF3 NP's (0,1 g/L) in DMEM+FBS. g, h Gemiddelde hydrodynamische straal (MHR) met standaarddeviatie van PrF3 en LaF3 NP's in DMEM+FBS bij verschillende concentratieniveaus
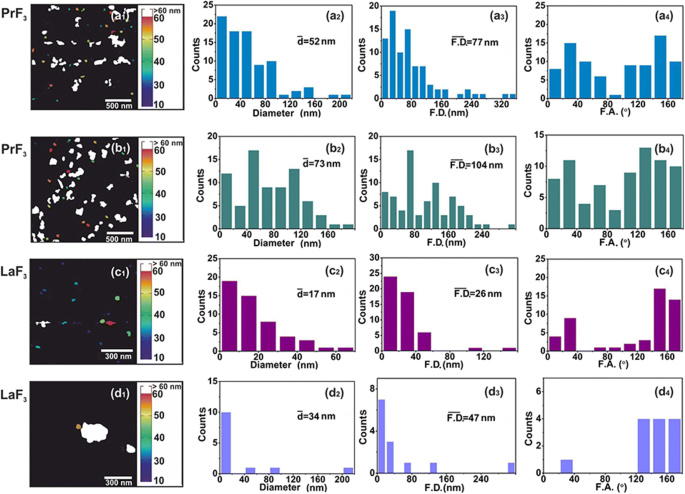
AFM digitaal (x , j ) groottehistogrammen van RE-NP's. (a1–d1 ) PrF3 (2 × 2 μm 2 ) en LaF3 (1 × 1 μm 2 ) NP's in DMEM+FBS-suspensies. (a2-d2 ) (x , j ) groottehistogrammen van gemiddelde gelijke cirkeloppervlaktediameter \( \left(\overline{d}\right) \) van RE-NP's. (a3-d3) (x , j ) groottehistogrammen van Feret-gebiedsdiameters \( \left(\overline{\ F.D.}\right) \) van RE-NP's. (a4-d4 ) Ferethoek (F.A.) histogrammen ten opzichte van de x -as. RE-NP's waren georiënteerd langs twee hoofdrichtingen tussen ± (44-60°)
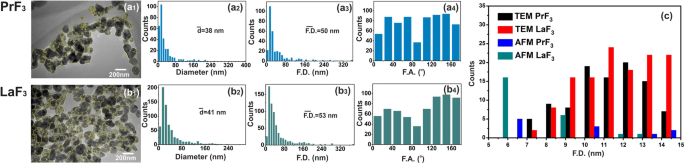
TEM digitaal (x , j ) groottehistogrammen van RE-NP's. (a1, b1 ) TEM-afbeeldingen van RE-NP's. Gele lijnen gaven 2D-grenzen tussen RE-NP's aan. (a2, b2 ) (x , j ) groottehistogrammen van gemiddelde gelijke cirkeloppervlaktediameter \( \left(\overline{d}\right) \) van RE-NP's. (a3, b3 ) (x , j ) groottehistogrammen van Feret-gebiedsdiameter \( \left(\overline{\ F.D.}\right) \) van RE-NP's. (a4, b4 ) Ferethoek (F.A.) histogrammen ten opzichte van de x -as met voorkeursrichtingen op ±(44-60°). (c ) Groottehistogram van Feret-diameter van kleine en kleine RE-NP's geëxtraheerd uit zowel AFM- als TEM-afbeeldingen voor 4 μm 2 gebieden
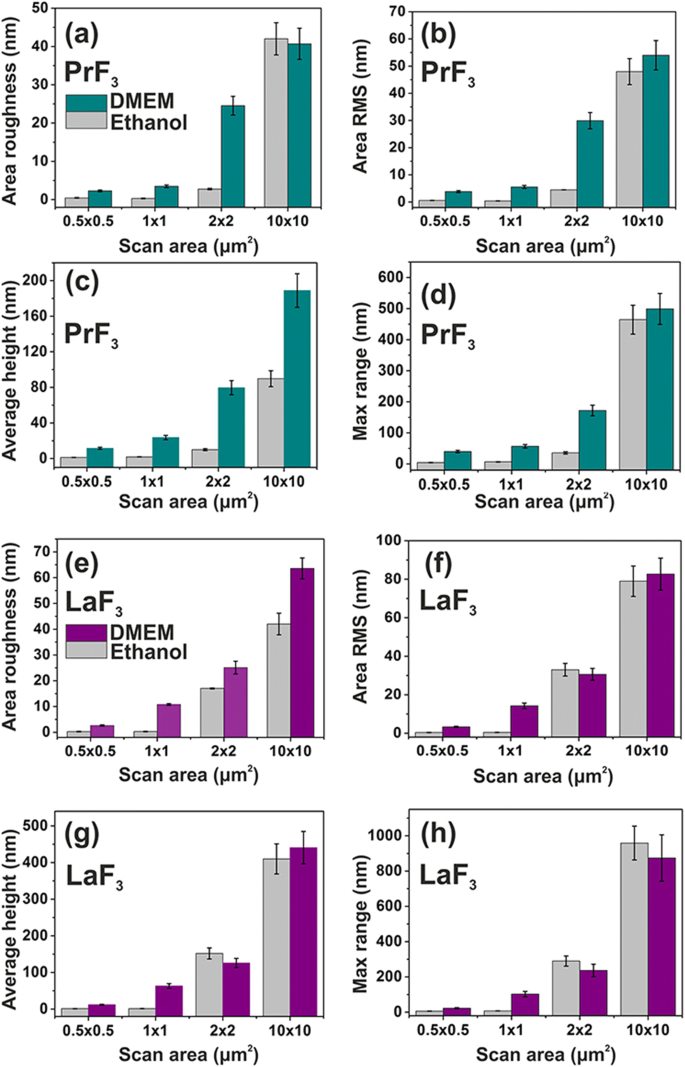
Oppervlakteparameterhistogrammen (z-hoogte) van PrF3 en LaF3 RE-NP's op een glassubstraat in DMEM + FBS en ethanol voor verschillende scangebieden. een , e Ruwheid van het gebied. b , v Gebied RMS. c , g Van gemiddelde hoogte. d , u Maximum z -bereik
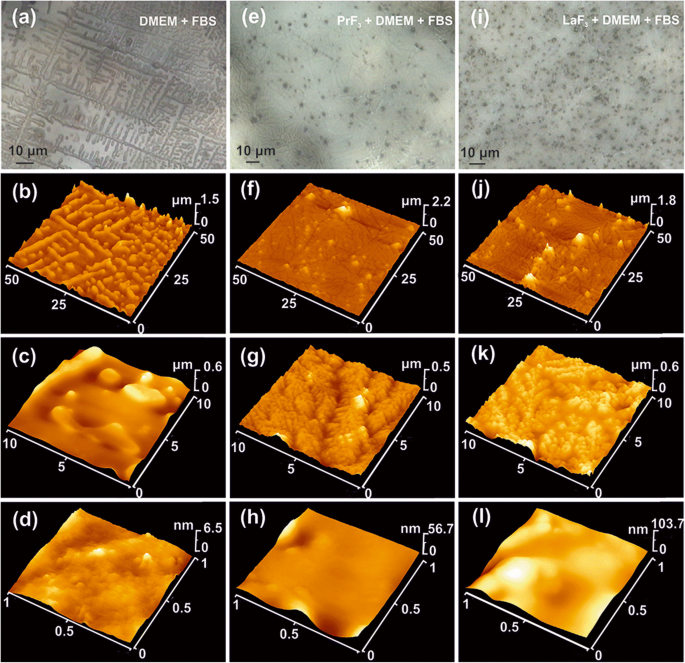
AFM en optische beelden van gedroogde RE-NP's in DMEM+FBS. een –d Optisch (a ) en AFM-beelden (b –d ) van DMEM + FBS met 5 m zelf-geassembleerde structuren. e –u CCD (e ) en AFM-beelden (f –u ) van PrF3 NP's in DMEM + FBS-media met 500 nm dendriet-zelf-geassembleerde structuren. ik –ik CCD (ik ) en AFM-beelden bij verschillende vergrotingen (j –ik ) van LaF3 NP's in DMEM+FBS met 100 nm dendriet-zelf-geassembleerde structuren
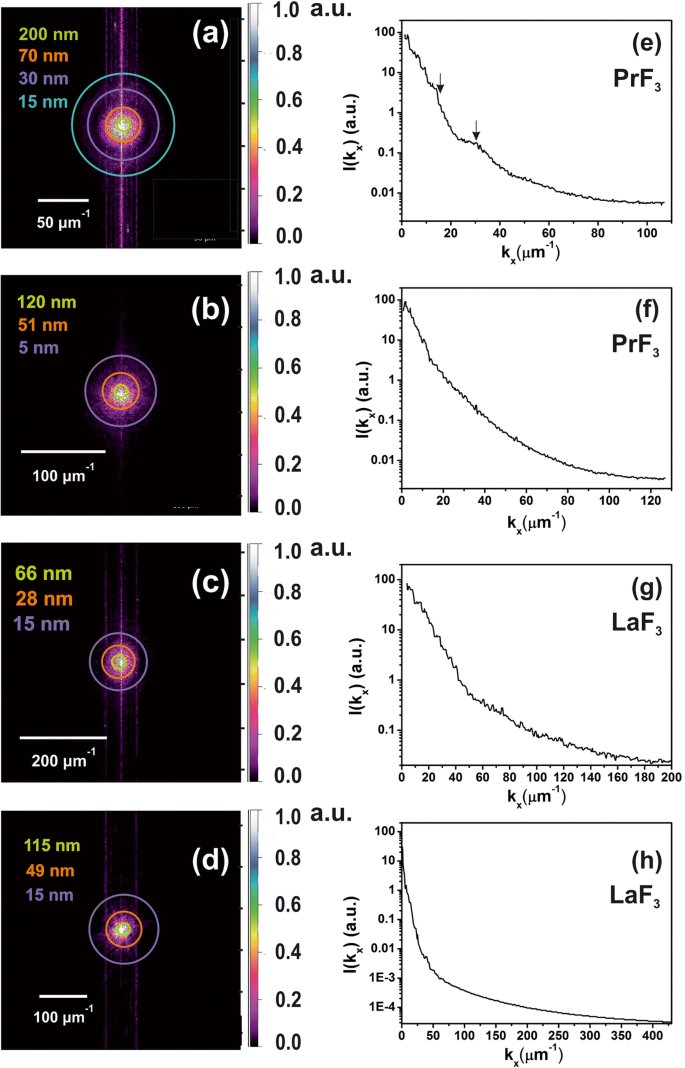
2D-FFT-spectra van z - hoogteverdeling van gedroogde RE-NP's in DMEM + FBS-media die de aanwezigheid van kleine NP's in de vloeibare suspensies aantonen. een , b z-hoogteverdeling van PrF3 NP's van AFM-afbeeldingen, Fig. 2 (a1, b1). Kleine z -hoogtekenmerken (~ 5 nm) werden geïdentificeerd in (b ). c , d z-hoogteverdeling van LaF3 NP's van AFM-afbeeldingen, Fig. 2 (c1, d1). e , v Vermogensspectra van z -hoogtegolfvectoren van PrF3 NP's langs de x -as. g , u Vermogensspectra van z -hoogte golfvectoren van LaF3 NP's langs de x -as
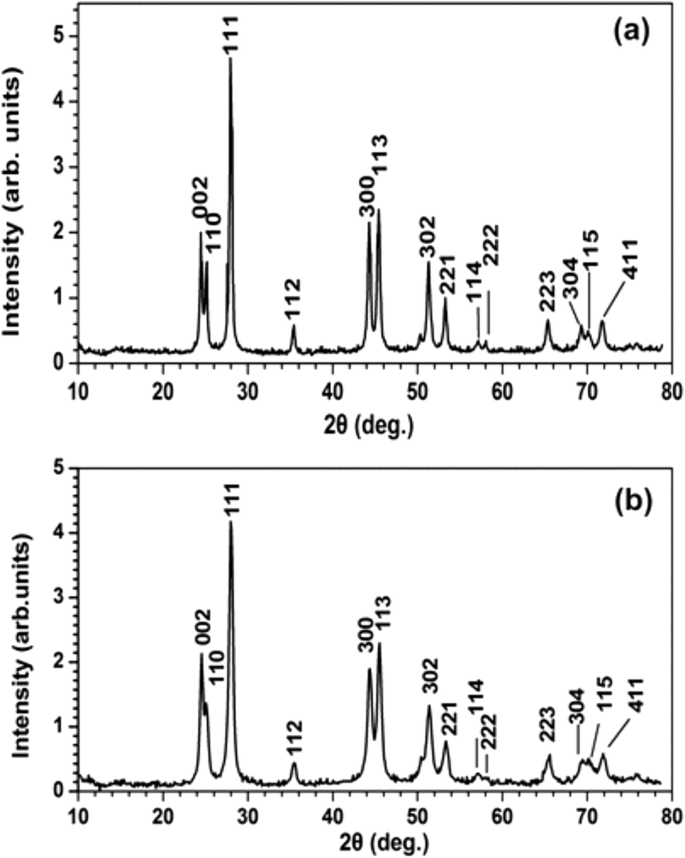
XRD-spectra van a PrF3 en b LaF3 NP's. De gemiddelde oppervlaktediameters van PrF3 en LaF3 NP's waren respectievelijk ~ 23 ± 4 en ~ 15 ± 4 nm
DLS
Bewolkte heterogene mengsels werden aanvankelijk gevormd door RE-NP's toe te voegen in celkweekmedia, wat de complexiteit van interacties tussen RE-NP's in vloeibare suspensies aantoont. Kleine (<-10 nm), kleine (>-10 en <-20 nm) en grote (>-20 nm) structuren van RE-NP's werden geïdentificeerd voor zowel PrF3 en LaF3 oplosbare stoffen (Fig. 1a-d).
De gemiddelde hydrodynamische straal (MHR) waarden van grote RE-NP's (55-83 nm voor PrF3 en 99–296 nm voor LaF3 ) werden direct gevolgd en omgekeerd evenredig met het concentratieniveau van NP's (0,1-10 kg m −3 ) in vloeibare Dulbecco's gemodificeerde Eagle's met foetaal runderserum (DMEM+FBS), Fig. 1g, h. Ook de MHR van kleine LaF3 en PrF3 NP's bleven constant, respectievelijk 10,66 ± 0,74 nm en 10,64 ± 0,40 nm bij verschillende concentratieniveaus van RE-NP's. De MHR van RE-NP's bleef ten minste 6 dagen onveranderd. Na het drogen van de RE-NPs-suspensies was het onmogelijk om het RE-poeder opnieuw op te lossen, omdat grote agglomeraties gestabiliseerd werden door sterke interacties die precipitatie forceerden.
AFM- en TEM-beeldvorming van RE-NP's en oppervlakteanalyse
Voor een betrouwbare grootteverdeling en statistieken van kleine RE-NP's in DMEM+FBS bij 0,1 kg m −3 AFM (scangebieden 1 × 1 en 2 × 2 μm 2 ) en TEM-beeldvorming werden ook toegepast (Fig. 2 (a1-d1) en Fig. 3 (a1, b1)). Na het overbrengen van de vloeistofdruppels van de RE-NP's in DMEM + FBS naar het glassubstraat, werd een relatief groot aantal niet-geaggregeerde kleine RE-NP's geïdentificeerd [38] uit zowel de gemiddelde grootte als de gemiddelde Feret-diameter van de NP's ( Fig. 2(a2-d2, a3-d3) en Fig. 3(a2, b2, a3, b3)). Ook gaven de histogrammen van de hoekverdeling van AFM- en TEM Feret-diameters (voor de grotere afmeting van NP's) aan dat beide RE-NP's bij voorkeur in twee richtingen waren georiënteerd tussen ± (44-62 o ), relatief ten opzichte van de x -as (Fig. 2(a4-d4) en Fig. 3(a4, b4)).
Ondanks dat z -hoogteverdeling van NP's gaf geen directe informatie over de totale grootteverdeling van NP's, het is een handig vergelijkend hulpmiddel voor de eerste schatting van (x , j ) grootteverdeling omdat de z-hoogte en (x , j ) distributies blijven met elkaar verbonden [38].
De gemiddelde oppervlakteparameters van zowel PrF3 en LaF3 in gedroogde suspensies voor verschillende AFM-scangebieden worden ook getoond in Fig. 4. De kleine z -hoogtewaarden gaven een zeer uniforme z . aan -hoogteverdeling van beide RE-NP's voor kleine 1 × 1 μm 2 gebieden scannen. Integendeel, voor zowel RE-NP's als grotere scangebieden, is de z - hoogteverdeling was significant breder. De lage z - hoogteverdelingswaarden bij kleine scangebieden weerspiegelen de aanwezigheid van kleine RE-NP's in de vloeibare suspensies. De waarden van de oppervlakteparameters in DMEM+FBS-medium waren gemiddeld groter dan in ethanol, en vertoonden een complexe reactieve toestand tussen eiwitten en RE-NP's, in overeenstemming met de veelzijdige structurering van Fig. 5 en de 2D-FFT-gegevens (Fig. 6). ). Over het algemeen LaF3 NP's vertoonden een intrigerende respons in gedroogde suspensies en uitgebreidere parameters voor oppervlakteruwheid dan PrF3 NP's.
FFT
Gekleurde ringen werden toegevoegd in geselecteerde stralen in de 2D-FFT-spectra (Fig. 6a-d). De cycli vertegenwoordigen verschillende NP's-grootteverdeling in de Euclidische ruimte, van een kleine grootte gelijk aan de grootte van een pixel (1,9-3,9 nm) tot een aanzienlijke grootte van ~-2 m, wat de bovenste scanlimiet was van de AFM-tip in de z -as (Fig. 6e-h). Een deconvolutie van de z -hoogtewaarden met de straal van de AFM-tip geven een werkelijke resolutie in de z - hoogteverdeling van ~ 5 nm. De 2D-FFT-spectra toonden een intense verdeling van golfvectoren nabij het centrum, als gevolg van een gemiddelde z -hoogte van RE-NP's van ~ -44 nm. De FFT-patronen vertoonden een halostructuur, die geleidelijk wordt uitgesmeerd, vanwege een polydisperse brede kleine structuur die is geïdentificeerd in de 2D-FFT-spectra. Omdat alleen de halo in de spectra verscheen zonder diffractieve patronen voor beide 2D-FFT-spectra, ontbraken reguliere zelf-geassembleerde structuren. De karakteristieke correlatielengtes verkregen uit de ringachtige 2D-FFT-patronen van PrF3 en LaF3 waren respectievelijk ~ 51, 70 nm en 28, 49 nm, in overeenstemming met de MHR-waarden geëxtraheerd uit de DLS-spectra.
XRD
XRD-spectroscopie karakteriseerde de kristalstructuur en leverde aanvullende informatie op over de grootte van PrF3 en LaF3 NP's (Fig. 7). De scherpe diffractiepieken, overeenkomend met de standaard hexagonale fasestructuur voor beide RE-NP's, onthullen een hoge kristallijne toestand van de agglomererende fasen. Door gebruik te maken van de Scherrer-formule (\( \tau =\frac{0.9\lambda }{\beta \cos \left(\theta \right)}\Big) \), wordt de gemiddelde gemiddelde cirkeldiameter (MEAC) τ van PrF3 en LaF3 NP's werden geschat op respectievelijk ~ 23 ± 4 en ~ 15 ± 4 nm.
VUV-spectroscopie
Het VUV-transmissiespectrum van de hydroscopische PrF3 NPs-laag afgezet op CaF2 substraat, van 125 nm (~-10 eV) tot 190 nm (~ -6.5 eV) wordt getoond in Fig. 8. De VUV-pieken bij 140-170 nm die eerder werden toegeschreven aan de overgangen van Pr 3+ driewaardige ionen uit de grond 4f elektronische statusconfiguratie voor de Stark-componenten van de 4f5d elektronische configuratie binnen YF3 , LaF3 , KY3 F10 en LiLuF4 eenkristalmatrix en ze zijn overlapt met een water-VUV-absorptieband, wat de aanwezigheid van gebonden watermoleculen in de PrF3 onthult en LaF3 kristallen.
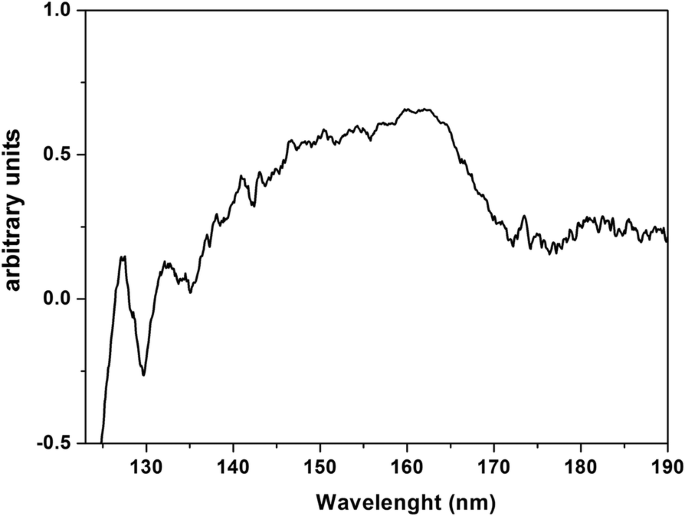
VUV-transmissiespectrum van PrF3 NP's in watersuspensies afgezet op de gedroogde CaF2 substraat. Het spectrum geeft wateraanhechting en wateropsluiting aan binnen PrF3 NP's
Levensvatbaarheidstest
Na analyse van de grootteverdeling en statistieken van RE-NP's, werd de levensvatbaarheidstest van wateroplosbare tetrazoliumzouten (WST) gebruikt om de toxiciteit van PrF3 te controleren. en LaF3 NP's voor drie menselijke kankercellijnen, de A549 afgeleid van longkanker, SW837 afgeleid van darmkanker en MCF7 afgeleid van borstkanker. Er werden drie verschillende concentraties RE-NPs-suspensies (0,5, 1 en 5 mM) in DMEM+FBS (A549, SW837) en Roswell Park Memorial Institute-medium met foetaal runderserum (RPMI+FBS) (MCF7) gebruikt. De cellijnen werden aanvankelijk op platen met 96 putjes geplaatst, die men een nacht liet hechten. Om binnen het lineaire gebied van celgroei te zijn en verzadiging een dag later te voorkomen (Fig. 9a), vers medium dat PrF3 bevat en LaF3 suspensies werden toegevoegd en levensvatbaarheidstests werden 24 en 48 uur later uitgevoerd na de toevoeging van RE-NP's, of 48 en 72 uur na het eerste moment van celplating. Voor de drie concentraties en de drie kweekcellijnen werd echter een overgroeiverschil gedetecteerd, op voorwaarde dat het medium niet werd vervangen en er geen extra RE-NP's aan de kweek werden toegevoegd, praktijken die de beginomstandigheden van het experiment veranderen. Het was ook onmogelijk om een celconcentratie van minder dan ~ 5 × 10 4 te plateren. cellen per putje omdat de samenvloeiing voor de drie cellijnen te klein was om een meetbare celgroei te garanderen. De optimale experimentele opstelling was opgezet voor ~ 5 × 10 4 cellen per putje.
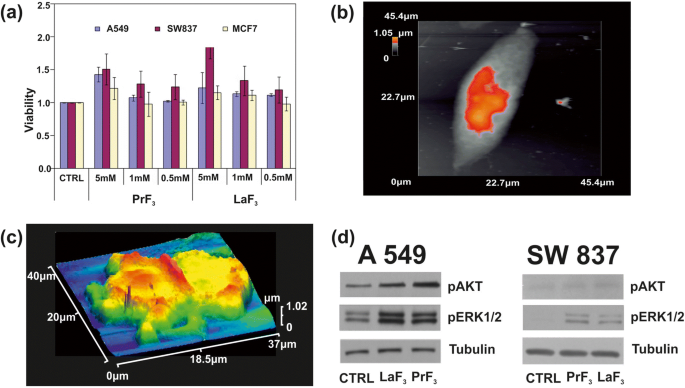
een WST-levensvatbaarheidstesthistogrammen van drie verschillende kankercellijnen (A549, SW837, MCF7) behandeld met verschillende concentraties PrF3 en LaF3 NP's in biologische media. b AFM-beeld van een enkele A549-kankercel. c AFM-beeld van een verdeelde A549-kankercel in RE-NP's in DMEM + FMS. d Wb-fosforylatieanalyse van de A549-, SW837-cellen met AKT- en ERK-routes
Bij hogere concentratie (5 mM) werd voor beide RE-suspensies een stijgende groei voor alle cellijnen verkregen (Fig. 9b, c). Onder hen was de hoogste groeiwaarde voor de SW837-lijn (86%, LaF3 ). Minder uitgesproken, maar nog steeds relevant, werd een celovergroei (15%) opgemerkt voor de MCF7-cellijn bij 5 mM. Een t test statistische analyse (p en Fisher F waarden) van de levensvatbaarheid van tumorcellen toonden aan dat de groei van tumorcellen na 24 uur onverzadigd was; het volgde een onbekende fysieke wet die de levensvatbaarheid en concentratie van RE-NP's verbindt (aanvullend bestand 1).
Fosforyleringsassays
De fosforyleringsstatus van twee eiwitten werd ook getest (Fig. 9d). Met behulp van specifieke antilichamen en Wb-assays in de A549- en SW837-cellijnen groeide in DMEM+FBS met 5 mM LaF3 en PrF3 NP's gedurende 24 uur, hoge fosforyleringsactiviteit van ERK1/2 en AKT in behandelde cellen, in vergelijking met controlecellen (CTRL), werd verkregen.
Discussie
De relatieve groeisnelheid van kankercellen nam toe bij hogere concentratieniveaus van beide RE-NP's (Fig. 9a). De MHR-waarden van RE-NP's in DMEM+FBS werden echter direct gevolgd (PrF3 ) en omgekeerd evenredig (LaF3 ) tot de concentratie van RE-NP's van 0,1–10 kg m −3 (Fig. 1g, h). Daarom zouden RE-NP's met een gemiddelde grootte van meer dan ~ 55 nm geen enkel effect moeten hebben op de celgroei en alleen kleine NP's kunnen een daadwerkelijke rol spelen in tumorgroei.
Grootte en structuur van RE-NP's
Identificatie van kleine RE-NP's
Uit de experimentele gegevens werden de gemiddelde grootte, distributie en de statistische parameters van RE-NP's geëxtraheerd. Door t . toe te passen teststatistieken voor de "nulhypothese" van "gemiddelde gelijke oppervlaktecirkel" van NP's in gedroogde PrF3 en DMEM+FBS-schorsingen, de p waarde van de diameter van NP's tussen twee willekeurig geselecteerde AFM-afbeeldingen was ~ 0,001 (aanvullend bestand 2). Een waarde van de MEAC-diameter (63 nm) werd met vertrouwen uit de AFM-gegevens gehaald en dit was vergelijkbaar met de MHR-waarde uit de DLS-gegevens (Fig. 1g).
Integendeel, de waarde van de MEAC-diameter van willekeurig geselecteerde LaF3 monsters vertoonden een gemiddelde MEAC-diameter van 26 nm met een hogere afwijzingswaarschijnlijkheidswaarde (p = 0.07), wijzend op een afwijkend gedrag van LaF3 in vloeibare suspensies. De discrepantie tussen de MEAC-diameter en de MHR van DLS (296 nm) (Fig. 1) is te wijten aan de complexiteit van interacties in LaF3 weer schorsingen. Inderdaad, voor een 2 × 2 μm 2 AFM tip scangebied, de gemiddelde z -hoogte was ~ 140 nm, wat de aanwezigheid van groot formaat LaF3 . weergeeft NP's, overgebracht van de vloeibare suspensies op het substraat (figuur 4). Voor de "nulhypothese" van "gelijke MEAC-diameterwaarden van willekeurig geselecteerde TEM-monsters", de p waarden waren ook klein (p = 0,001). Voor beide RE-NP's zijn de gemiddelde MEAC-diameterwaarden geëxtraheerd uit de gecombineerde TEM- en XRD-gegevens voor beide PrF3 en LaF3 aangegeven hoge p waarden, p =0,29 en 0,06, waardoor er geen correlatie tussen de TEM- en XRD-gegevens mogelijk is. Alleen TEM, AFM (PrF3 ) en DLS-gegevens waren voldoende betrouwbaar om de MEAC-diameter en core-shell-waarden te extraheren (aanvullend bestand 2).
Ook gaf een niet-isotrope hoekverdeling van Feret-diameters aan dat zowel PrF3 en LaF3 structuren waren sterk gepolariseerde diëlektrica, omdat de anisotrope hoekverdeling een indicatie is van sterke elektrische polaire interacties tussen nanokristallen. Een diverse gepolariseerde toestand van LaF3 was verantwoordelijk voor het verlagen van de relatieve efficiëntie van de agglomeratietoestand in suspensies en het opschalen van de oppervlakteruwheidsparameters in gedroogde monsters.
Een softwaredeeltjesanalyse van willekeurige AFM-beelden voor 5 μL en een concentratie van 0,1 kg m −3 identificeerde een aantal ~ 22 en ~ 11 RE-NP's met een grootte kleiner dan 15 nm en 10 nm (p = 0,001), en een aantal ~ 60 RE-NP's van TEM-beelden (p = 0,001) in een gebied van ~ 4 μm 2 , bevestigend dus de aanwezigheid van kleine RE-NP's in de suspensies (Fig. 3(c), Aanvullend bestand 2) niet gedetecteerd met DLS.
Structuur en geometrie van RE-NP's
De grootteverdeling van RE-NP's is divergent in ethanol- en DMEM + FBS-suspensies (figuur 4). De diversiteit is het bezit van verschillende moleculaire interacties tussen de geadsorbeerde eiwitten, koolhydraten, elektrolyten en het oppervlak van RE-NP's, wat leidt tot de vorming van zeer complexe organische mantels (corona), die de specifieke interacties van RE-NP's met cellen in DMEM+FBS-medium.
Het samenspel tussen watermoleculen gevangen in hygroscopische RE-NP's en DMEM + FBS was ook van vitaal belang voor de vorming van kernschil. Het had ook een diepgaand effect op eiwit- en conformationele veranderingen in de tussenliggende interacties tijdens de beginfase van de voorbereiding. Omdat de oppervlakte-tot-bulkverhouding van NP's hoge waarden in de suspensies ontwikkelde, waren de effectieve stabiliteit en de fysisch-chemische, mechanische en stroomeigenschappen van RE-NP's, inclusief het vermogen om eiwitten te absorberen, buitengewoon gevarieerd [39,40,41] .
Vergelijkende grootteverdeling van RE-NP's in vloeistof (DLS) en gestolde suspensies via AFM en TEM toonde aan dat RE-NP's waren ingekapseld in organische vormen die diëlektrische kern-schaalstructuren vormden, waar een eiwitachtige schaal de RE-kern omringt. De AFM afgebeeld van gestolde RE-NP's in DMEM + FBS-suspensies die zijn afgezet op glassubstraten, wijzen ook op de vorming van veelzijdige RE-NP's en eiwitcoronacomplexen (Fig. 5). Terwijl gedroogde media een regelmatig zelf-geassembleerd patroon van kristalstructuren vormden (Fig. 5a-d), vertoonden gedroogde RE-NP's-suspensies een amorfe gelaagde structuur met verschillende zwarte vlekken, zelfs zichtbaar met de digitale camera van de AFM (Fig. 5e-l) . Bij hogere optische vergroting werden discrete agglomeraties van bolvormige vormen, kleiner dan die in het medium alleen, ook gedetecteerd voor beide RE-NP's in DMEM + FBS, samen met dendrietachtige structuren, die beide de complexiteit van interacties laten zien, in overeenstemming met de oppervlakteparameterresultaten (Fig. 4). Zelfs met de hoogste AFM-resolutie (1 × 1 μm 2 gebied), de laatste baan van Fig. 5, werden geen geïsoleerde RE-NP's-aggregaties, binnen de resolutielimiet van ~ -5 nm, geïdentificeerd in gedroogde structuren voor beide RE-NP's. De zwarte bolvormige mycelia, 1-2 m lang, getoond in de optische afbeeldingen, waren grote agglomererende formaties van core-shell RE-NP's. De complexiteit van reacties tussen de RE-NP's en DMEM+FBS werd gevisualiseerd via de transformatie van de zelf-geassembleerde langwerpige structuren op lange termijn in pure DMEM+FBS naar dendrietstructuren.
De resultaten wijzen op het beeld van een enkele RE-NP-kernstructuur ingekapseld in een eiwitomhulsel. Deze structuren waren niet detecteerbaar omdat ze waren omgeven door organisch materiaal en elektrolyten, die beide een kruisreactie vertoonden met RE-NP's. Het VUV-spectrum van PrF3 toont enkele spectrale pieken tussen 140 en 170 nm (Fig. 8). De ionische overgangen worden overlapt door een VUV-waterabsorptieband die is verlengd van 145 tot 180 nm met een maximum bij 168nm. Alleen de spectrale handtekeningen van de 4f6s elektronische configuratie met maxima bij 132 en 127 nm waren in het spectrum aanwezig. However, these bands could evince the presence of water in the high hygroscopic PrF3 suspensions. Water has a rich, structured absorption band in the VUV spectral range centred at 122 nm, revealing the presence of water molecules in the core-shell NPs.
Activation of Mechanosensors
Activation of Integrins by External Forces
The activation of oncogenic pathways by RE-NPs [24], besides the 3D structural nature of TSRs, is based on some Natural Evolution principles for sustaining the viability of cells. First, upon binding a specific external ligand in a LABS, conformational changes along the entire TSR spectrum underline a series of cascading pathways, triggering tumour cell growth (Fig. 9c, d). The transmission of signals advances through the plasma membrane via various protein chains. Signal transduction was via conformational transformations of integrins responding to a high affinity external force (Fig. 10a, b).
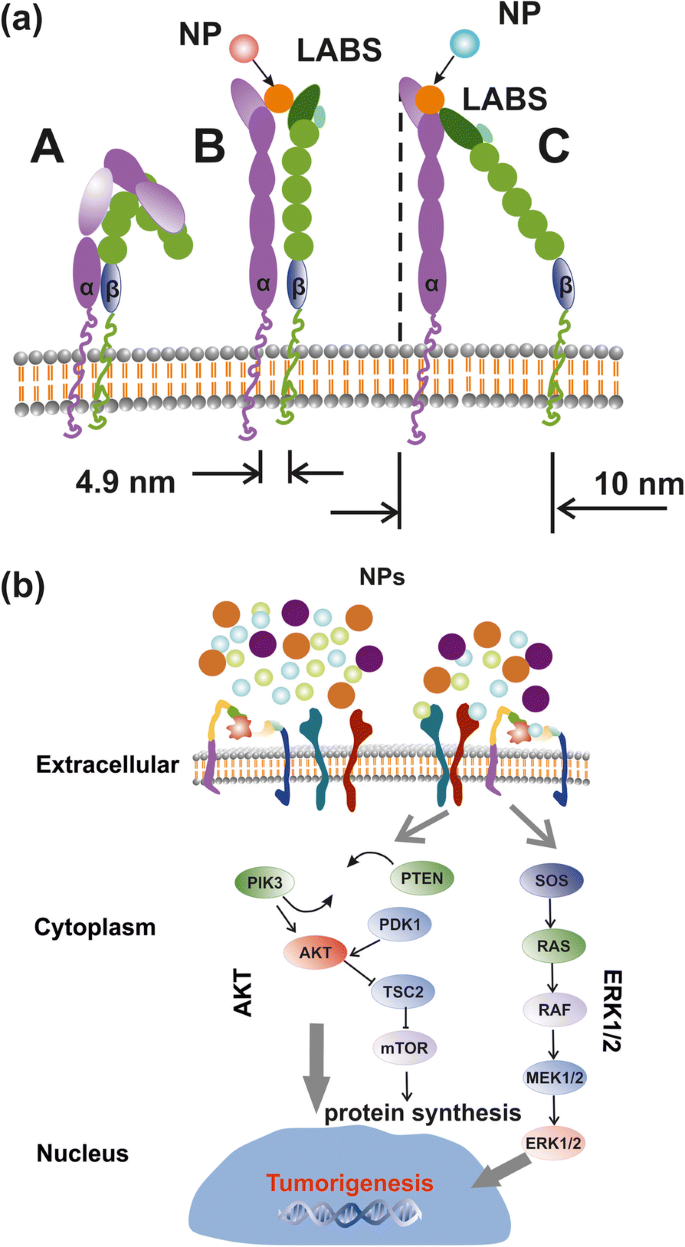
Simplified layout of integrin activation by NPs and signal transaction pathways. een Structure and conformational geometries of integrins at a low (A), medium (B) and high-affinity strengths (C). b AKT and ERK1/2 signal transaction pathways activated by RE-NPs via external integrin stimulation
Because of “life sustainability” and “survival laws” that prevents cancer cell growth by random “noise”, it is required that the strength of the external force should be within a bounded range of values and also the external strength stimulus should apply for a long period on a large number of mechanosensors in a cancer cell. The external strength that stimulates cancer must be slightly larger than the strength of the interatomic molecular forces under normal conditions. For a thermal energy of a ligand at room temperature (kT = 0.025 eV, T = 298 K), and for a regular thermal stress of molecular bonds of ~ 0.05 nm, the mean thermal force acting on the LABS stays for 1.2 × 10 −12 N. In principle, a force above ~ 10 × 10 −12 N acting coherently on the whole set of mechanosensors on a cell should activate signal transduction in tumour cells. Consequently, ignoring any thermal and mechanical stressing in the ECM normal conditions, integrin activation via electrical polar interactions between LABS and NPs has the potency to start signal transduction in cancer cells and to initiate tumorigenesis.
Integrin Structure and Geometry
An integrin receptor in the upright conformation state extends ∼ 20 nm upwards from the cell membrane [42] (Fig. 10a). For no contacts between the two α- and β-subunits, other than those in the headpiece near the ligand-binding pocket, the α- and β-subunits are well separated with their cytoplasmic tails extended out up to ∼ 8 nm [42]. A conic projection geometry (20 nm slant height, 5–10 nm diameter of its circular base), bounded by the α- and β-subunits, defines a projected area on the surface of cell’s membrane between ~ 19 and ~ 80 nm 2 , for a typical mean radius of a tumour cell R c ≈ 5 μm (equivalent surface area of a spherical cell \( {S}_c=4\pi {R}_c^2=3.14\ \mathrm{x}\ {10}^8\ {\mathrm{nm}}^2 \)). By dividing the area S c of a spherical tumour cell surface with the projected area of an integrin on a cell surface, an upper limit of the number of integrin receptors for these projected areas was n int = 1.6 x 10 7 and 3.9 × 10 6 respectievelijk. These numbers are compared with the mean number of integrins on a cell \( {\overline{N}}_{int}\approx 2\ \mathrm{x}\ {10}^5 \) and for an average interspacing of 45 nm between adjacent integrin receptors [43]. Nevertheless, \( {\overline{N}}_{int} \) might be larger because of an uneven surface structure, different separating distances between integrins and variable size of tumour cells (Fig. 9c), but the number of integrins on a cell membrane stand between n int and \( {\overline{N}}_{int} \).
Interaction of Mechanosensors with RE-NPs
ERK ½ and AKT Activation
The TEM images and the elemental mapping of F, La and Pr showed that RE-NPs were unable to penetrate inside the cell. They gathered around the A549 cell membrane (Fig. 11), confirming that an external force can stimulate cell growth because of TSRs activation [44]. The Pr atoms were distributed around the boundaries of the cell’s membrane. The small numbers of F, La and Pr identifications inside the cell were not associated with endocytosis of RE-NPs, but they were images of RE-NPs from the projections of the two cells hemispheres on cell’s equatorial cycle.
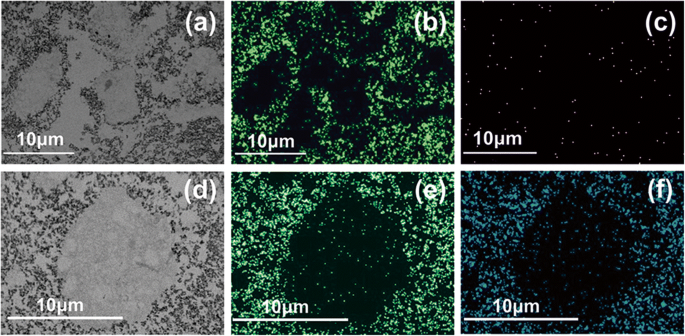
TEM images and elemental analysis of RE-NPs at the surface boundaries of A549 cells. een TEM image of small size LaF3 NPs surrounding the A549 cells. b Elemental analysis of F atoms in RE-NPs distributed around the cell. c Elemental analysis of La atoms. The low concentration of La atoms was associated with a rather small scattering efficiency of the X-rays. d –f The same as for (a –c ) for PrF3 RE-NPs
It was also evident that both RE-NPs were able to enhance AKT phosphorylation, especially in A549 cells (Fig. 9d), where the steady-state level of AKT pathway activity was higher for the SW837 cell line. The phosphorylation level for the MCF7 cell line was below the detection limit, in agreement with the relatively low levels of growth. High phosphorylation levels of ERK1/2 [36] and AKT were detected in A549 and SW837 cell lines. Cell growth was started once NPs with a proper size interact with the mechanosensors of the cells to provide the correct force for initiating cell growth [45, 46]. ERK and AKT pathways were frequently active in several cancer cell types via extracellular springing, as they were stimulated by the TSRs, upon a selective binding with various mitogenic ligands, or via the activation of the mechanosensory group. The interaction was responsible for a continuous intracellular stimulation that, according to the cell’s phenotype, driven the cancer cells to uncontrolled and endless growth. Viability tests were also run for 48 and 72 h, but the growth of all cell lines was saturated at 48 and 72 h after the initial moment of Cell plating.
Interaction of Cells with Ions
Likewise, as fluoride anions are the most reactive electronegative elements and, the mean radii extension of the unscreened 4f electronic configuration of La and Pr trivalent ions are relatively large, high electric surface charges could be developed via electric dipole interactions [47].
One crucial question stands whether a single ion binding on a specific site can activate tumour cell growth. Because the projected area of the 4f electronic configuration of a single RE ion is S 4f = 0.040 and 0.043 nm 2 (for an approximated spherical geometry of the 4f electronic configuration and a 4f mean orbital radii ~ r 4f =0.113 and 0.117 nm for Pr and La ions, respectively), a typical upper limit number of single RE ions, or other equivalent size ions, over the whole area of the cell membrane was ~ Sc / S 4f = N 4f ~7.9 × 10 9 RE ions; a number which is at least two orders of magnitude above the upper limit of the mean number of integrins on a tumour cell. As the relative overgrowth of cells was ascending with rising concentration (Fig. 9a), it is unlikely that tumour cell growth is triggered by a specific binding of single trivalent RE ions [48] on the ligand sites [49,50,51]. Indeed, the large number of RE ions should have saturated the cell’s growth and thus the viability of cells should have remain independent from the concentration of the RE ions.
Interaction of Integrins with RE-NPs
Within the requisite force range of few pN, and for efficient activation of integrins from NPs, the interaction between NPs and LABS should activate a large fraction of integrins of the cell for a long time. In the most extreme favoured case for cell growth, the number of NPs had to remain equal with the number of integrins on the cell’s surface, and the interactive force between LABS and NPs has to be attractive for obtaining a constant (long-term) action. A thin spherical shell of spherical NPs surrounding a tumour cell occupied a volume\( {V}_{sc}\approx 4\uppi {R}_c^2x \), where R c = 5 μm is the cell radius and x ≈ 20 nm is half the separating distance between adjacent integrin receptors and V sc ≈ 6.3 x 10 9 nm 3 . For justifying the requirement that each integrin receptor interacts only with one NP, a first estimation of the size of NPs to meet the above requirements for the whole set of integrins on a cell is obtained by dividing the volume of the spherical shell V sc with the number of integrins. A simple calculation for a cell radius 5 μm shows that the limits of radii of NPs activating the whole set of integrins within the spherical shell volume V sc ≈ 6.3 x 10 9 nm 3 covering the cell is obtained by divided the volume V sc with the number of integrins \( {\overline{N}}_{int}\approx 2\ \mathrm{x}\ {10}^5 \) and n int ≈ 1.6 x 10 7 . The volume of the spherical NPs stands for 3.15 × 10 4 and 3.93 × 10 2 nm 3 respectievelijk. Therefore, the radii of the NPs interacting with an integrin lay between ~ 20 and 5 nm. Allowing for one order of magnitude variations in the number of integrins \( {\overline{N}}_{int} \), the radii of the NPs interacting with integrins is between ~ 27 and ~ 3 nm respectively.
By also applying similar simple calculations and within the experimental limits of concentration levels of RE-NPs (0.1–10 kg m −3 ), the maximum numbers of PrF3 with MHR 55–83 nm and LaF3 with MHR 296–100 nm NPs (Fig. 1g, h) covering the surface of a tumour cell V sc stood for 4.1 × 10 4 –2.1 × 10 4 and 17.1 × 10 2 –1.5 × 10 4 NP's. These values are placed well below the number of integrins on the cell surface. For rising concentrations of PrF3 and LaF3 from 0.1 and 10 kg m −3 , the number of PrF3 and LaF3 NPs in the suspensions must go up for either descending or ascending size of NPs. As viabilities of cancer cells are raised at higher concentration levels, it is unlikely that 55–296 nm sized RE-NPs are responsible for cancer cell mitosis under the current experimental configuration.
Also, from the DLS data, the size of both RE-NPs between 10 and 20 nm remained constant (10.6 nm) at different RE concentrations. The number of RE-NPs with this size covering the cell surface is between 3.7 × 10 5 and 1.5 × 10 6 . This number is comparable with the mean number of integrins \( {\overline{N}}_{int}\approx 2\ \mathrm{x}\ {10}^5 \) on a cell surface. Therefore, only small size RE-NPs have the potency to stimulate cancer cell growth by stimulating all the integrins on a cell surface, in agreement with the experimental observations (Figs. 1g, h and 9a).
The number of tiny sizes RE-NPs with MEAC diameter (TEM) from 2 to 10 and 10 to 15 nm on the cell surface (S c = 314 μm 2 ) stands for 1.3 × 10 4 and 1.8 × 10 4 RE-NPs, respectively. Those values stayed one order of magnitude below \( {\overline{N}}_{int}\approx 2\ \mathrm{x}\ {10}^5 \) and therefore tiny size RE-NPs had also the potency to justify the experimental results of rising viability values with concentration (Fig. 9a). Also, the rough surface of tumour cell (Fig. 9c) is able to form cavities, where small size RE-NPs are trapped, triggering thus cell’s mechanosensors. Most important, only tiny size RE-NPs have the potency to activate integrin receptors via electrical dipole interactions (vide infra).
Interaction of EGFR with RE-NPs
An upper limit of small size NPs capable of stimulating cell’s overgrowth via the EGFR was set previously to 14 nm [52], but a realistic size of NPs stimulating the EGFR should be < 5 nm [53] (Fig. 12). The area number density of EGFR on the surface of tumour cells stands for ~ 1.4 × 10 −4 nm −2 and the total number of EGFR on the surface S c of cells remains between ~ 4.2 x 10 4 and 10 5 [54,55,56]. RE-NPs with 5–10 nm size stayed for a number of 34 NPs (Fig. 3). Extrapolating this number to the surface of a cell S c , the total number of RE-NPs remained at ~ 10 4 NPs, a number which matches the number of EGFR receptors on a A549 cell. Therefore, the EGFR have the potency to be activated synergistically also by a number of tiny size RE-NPs.
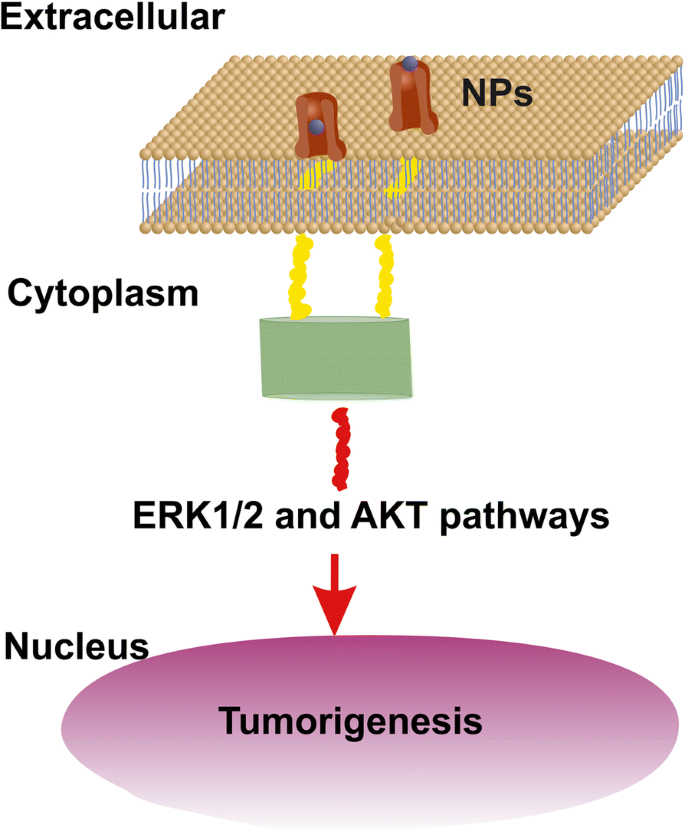
AKT and ERK1/2 signal transduction pathways activated by RE-NPs via EGFR stimulation. EGFR is activated only by tiny size ~ 5 nm NPs
Electric Dipole Interaction Between RE-NPs and LABS
The above experimental results are supported by the hypothesis of cancer cell growth from LABS stimulation by tiny size core-shell RE-NPs via electrical dipole interactions, Appendix.
Indeed, the mean electrical dipole force \( \left\langle {\overrightarrow{F}}_{V_2}\right\rangle \)acting on LABS from a core-shell RE-NP includes two terms (Fig. 13d and Appendix, Eq. A22). The first radial term is inversely proportional to the forth power of separating distance r 1 between the RE-NPs and LABS and is also proportional to the size of NP. The second polar term is inversely proportional to both the separating distance r 1 and the square power of the size of NP,
$$ \left\langle {\overrightarrow{F}}_{V_2}\right\rangle =-\frac{G_1{N}_2{N}_1\ d{e}^2}{4{\varepsilon}_0\ {r}_1\ }\theta \left(\ 3G\frac{b}{r_1^3}{\widehat{r}}_1+\frac{\theta }{2{b}^2}{\widehat{\theta}}_1\right)\kern0.75em (1) $$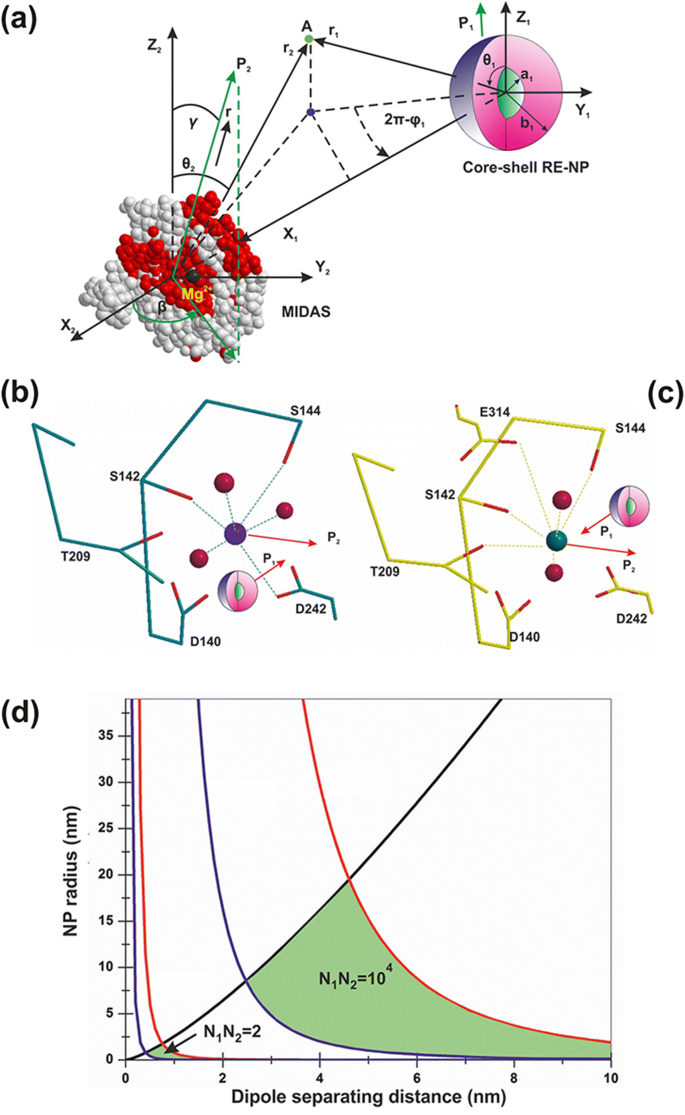
een Electrical dipole interaction between one core-shell RE-NP and one LABS. b , c RE core-shell NP near a MIDAS (b ) and ADMIDAS (c ) adhesion sites. d Locus area (green) of the size of RE-NPs and separating distance between a LABS and a core-shell RE-NP for two electrical charging states
In verg. 1, G en G 1 are the geometrical factors of NPs, describing either core-shell or core spherical structures, Appendix, Eqs. A6 and A14; N 1 , N 2 are the numbers of surface electrons on the a RE-NPs and LABS surfaces; d en b are the effective characteristic spatial extension of atomic orbitals of LABS, ~ 0.1 nm, and the radius of RE-NP; e en ε 0 are the electron charge and the vacuum permittivity and \( \theta =\frac{d}{r_1}<0.01\ rad \). Because the core of the RE-NPs is a crystalline semiconductive material, an inherent large number of surface and volume defective sites were accountable for a high density of pseudo-electron energy levels that allowed the electrons to move freely within the core volume [46]. Consequently, a core-shell structure had the potency to be highly polarised. Therefore, LABS can be activated efficiently by core-shell RE-NPs via electrical dipole interactions at close separating distances. The high polarised efficiency of the core nucleus was confirmed experimentally via the selective orientation of NPs along two distinct directions (Fig. 2(a4–d4) and Fig. 3(a4, b4)).
The polar interaction force is also proportional to the geometrical factor G 1 , Appendix, Eq. A14. Typical values of dielectric constants of the culture media, shell configuration and RE core components stand for ε 1 = 78, ε 2 = 10 and ε 3 = 15. When the ratio of core-shell to core radii b/a sets within 1 and 50, the geometrical factors G , G 1 retain almost constant values (G = 0.2, G 1 = 0.01) and they are self-same for both a spherical core (b/a = 1) and a spherical core-shell. Any permanent or induced polarisation of an open or closed a-I-MIDAS domain forming the LABS domain has its origin on six coordinated water oxygen atomic orbitals with Mn 2+ or Mg 2+ ions, arranged in a spherical geometric configuration [7] (Fig. 12a–c).
As the electrical dipole force in Eq. 1 stands for the vector sum of a radial (first term) and a polar component (second term), the last term prevails over the first one provided that
$$ {r}_1>\sqrt[3]{6G}b\sim b\kern0.75em (2) $$In this case, a LABS is activated from the polar force component for all (b/a) ratios and, most important this term is inversely proportional to the second power of the size of NPs, in agreement with the experimental results that only tiny or small size LaF3 NPs activated cancer cell proliferation.
The prevailed polar force term for different r 1 en b values and for different Ν 1 , Ν 2 charging states activating the LABS/MIDAS stay within the limits [57,58,59,60].
$$ {10}^{-12}N<\frac{G_1{N}_2{N}_1\ d{e}^2}{8{\varepsilon}_0\ {r}_1{b}^2\ }{\theta}^2<{10}^{-9}N\kern1em (3) $$Inequality 3 relates the size b of the RE core-shell NPs, the separating distance r 1 and the number of the bound or free electrons Ν 2 , Ν 1 on the surface of the two dipoles. The locus of points (r 1 , b ) satisfying the inequality 3 for different surface charge states Ν 1 , Ν 2 is bounded by the black, red and blue lines (Fig. 13c). As there was no specific assumptions for the type of RE-NP, results can be equally applied for any type of polarised NPs.
When the algebraic product of the number of the surface electrons N 1 en N 2 (bound or free) on the LABS and the RE-NP, respectively, was N 1 N 2 = 2, the locus of RE-NPs size and separating distance for integrin activation was < 1 nm. At higher charging states, N 1 N 2 = 10 4 , the locus area spans a wider RE-NPs size and separating distance area set of values, from 0.5 nm–19 nm to 2.5–15 nm, respectively.
From the above analysis, it is found that only tiny or small size NPs can activate LABS at a certain separating distance r 1 and the electrical dipole interaction strength decays inversely proportional to the second power of the size of NPs. From Fig. 13c and for a charging state with N 1 N 2 = 5 x 10 4 , the size of NPs capable to activate LABS is bounded by the limits
$$ 2.5\ \mathrm{nm} Most important, from Fig. 13c, both the locus area (green area) and the size of RE-NPs increase for higher electrical charging states.Conclusies
Cancer is a complex disease. Tumours are highly heterogeneous, and cell growth, among other factors, depends on dynamical interactions between cells and the continually changing extracellular matrix. Besides random genomic mutations, signal transductions in cells, activating cell growth can be triggered by mechanical, thermodynamic and electrical polar interactions between the microenvironment of the extracellular cell matrix and the membrane’s mechanosensors. Here, we demonstrated that tumour cell proliferation in three different human cancer cell lines (A549, SW837, MCF7) had the potency to be activated by a synchronised and synergetic activation of EGFR or via electrical dipole interactions between tiny size RE-NPs and the LABS of integrins on a cell.
Because the prerequisite force for integrin activation should stand between 10 −12 and 10 −9 N, the size of the active RE-NPs causing cell growth should be within certain limits. Cancer activation is specified by both the electrical surface charges on the LABS and the NPs and by their separating distance. This electric dipole activating force follows an inversely proportional square power law of the radius of NPs, evidencing that only tiny or small size RE-NPs have the potency to stimulate cancer cell growth via electrical dipole interactions, in agreement with the experimental results.
Methods
Synthesis of RE-NPs
PrF3 NPs were synthesised via co-precipitation. Briefly, 4 g of Pr2 O3 were added to 110 mL of 10% nitric acid in a polypropylene glass beaker together with 3 g of NaF under stirring. The mixture was heated to 50 °С and stirred for 45 min until a clear light-green solution appeared. Then it was filtered. The pH of the mixture adjusted to 4 by adding 25% of ammonium hydrate. Next, the mixture was stirred again for 20 min. Finally, the precipitated NPs washed with distilled water by centrifugation.
LaF3 NPs were also synthesised by applying the same protocol in a mixture of La2 O3 (4 g) and NaF (3 g). From both preparations, an aliquot of the suspensions containing NPs was air-dried for structural analysis and the remaining part kept as water suspension for the biological studies.
The suspensions of NPs were prepared in complete DMEM+FBS cell culture medium by adding water suspended NPs directly to the medium to a final concentration of 5 mM. Then, starting from the 5 mM stock solution, some subsequent dilutions using DMEM as a solvent were prepared to a final NPs concentration of 1 mM and 0.5 mM, respectively.
Size Distribution of RE-NPs
XRD
The crystal structure and the size of PrF3 and LaF3 NPs were characterised by XRD spectroscopy, with an X-ray diffractometer (Shimadzu XRD-7000S) in the 2θ range from 10° to 80° using the graphite monochromatised Cu-Ka radiation (1.5406 Å). The weighted average of τ for all peaks was used in the statistics. Weighting, besides β, took into account the relative intensity of every peak of the XRD spectra. The corresponding errors incorporate the reading error (0.3 mrad) and the standard error of the mean (se = σ / √ Ν ).
DLS
The size distribution and the MHR of RE-NPs in water and DMEM+FBS suspension were determined for comparison by DLS at 632.8 nm and right angles at 37 °C with a multi-angle dynamic and static light scattering instrument (PHOTOCOR-FC). The values of the MHR (Stokes radius) and the size distribution of NPs were calculated from the autocorrelation spectra and the Stokes-Einstein relation with the DynaLS software. Because the intensity of scattered light in pure DMEM+FBS was 20 times lower than with RE-NPs additives, the level of aggregating proteins in pure DMEM+FBS was negligible compared with mixed suspensions of RE-NPs in DMEM+FBS medium. MHR and RE-NPs size distribution and size errors were obtained by fitting and processing the data from the DLS instrument with the DynaLS software that allows the MHR to be calculated in different spectral domains of the main size distributions, from 10–10 2 to 10 2 –10 3 nm, Additional file 2.
AFM
Because size distribution below 15 nm was close to the low limit range of DLS, AFM was also applied to evaluate small size distribution. At low concentration of RE-NPs in liquid suspensions and slow drying rates of droplets on glass substrates, the deposits reflected the size distribution in the liquid suspensions [37]. Following the dispersion of RE-NPs in ethanol or DMEM+FBS, a drop of suspension was placed on a clean glass substrate using a micropipette, and then it was dried in air at room temperature for AFM imaging and analysis (diInnova, Bruker). AFM was performed in the tapping mode, in ambient conditions with a phosphorus-(n)-doped silicon cantilever (Bruker, RTESPA-CP), having a nominal spring constant of 40 nN/nm and operating at a resonance frequency of 300 kHz. Surface areas of various sizes (0.5 × 0.5–50 × 50 μm 2 ) were imaged with high spatial resolution (512 px × 512 px) at a scanning rate of 0.2 Hz to identify domains with different size distributions via “scan area filtering” [37]. From the morphological analysis by the SPM LabAnalysis V7 software, the particle’s size distribution, shape and aggregation stage were determined.
The size of NPs for different scanning areas was also noticeable by the particle analysis chromatic bar (Fiji integrated ROI colour coder based on MEAC diameter) (Fig. 2(a1–d1)). The AFM image was transformed into a binary image using an appropriate z -height threshold. Every pixel of the processed image contained information not only for the z -height in the pixel area but also for the presence of particles in the pixel area. De x -histograms of MEAC and Ferret diameter (Fig. 2(a2–d2, a3–d3)) were extracted by using the “Image J 1.51n Fiji distribution software”, with the correct z -height threshold values. The size resolution per pixel was 3.9 and 1.9 nm for PrF3 and LaF3 respectively.
The particle identification, the noise extraction and the particle area data were processed by the “Particle Analyser function” of Fiji software (Fig. 2(a1–d1)). The particle diameter histograms were also analysed. Both the equal area circle diameter (Fig. 2(a2–d2)) and Feret diameter or “calliper diameter” (maximum diameter of a particle among all directions) (Fig. 2(a3–d3)), whose direction was the Feret angle (Fig. 2(a4–d4)), were analysed. The mean equal area circle diameter and the mean Feret diameter were calculated taking into account all particles identified. The associated errors incorporated the actual pixel size in every AFM image and the standard error of the mean (se = σ / √ Ν ).
Een t test was performed for every set of AFM images based in the “null hypothesis” that the mean particle diameter was the same for all the AFM images between randomly selected figures (Fig. 2(a1–b1, c1, d1)). De p value (probability that the null hypothesis based on t distribution is not valid) is shown in Additional file 2.
TEM
The same technique was followed for calculating the above parameters in TEM imaging (Fig. 3(a1–b4)). Atomic resolution TEM (Hitachi HT7700 Exalens) imaged either extracellular or intracellular RE-NPs attachment on the A549 cells fixed in glutaraldehyde. Elemental analysis of F, La and Pr were also carried out (Oxford Instruments X-Max 80T).
2D-FFT
Additional information on the NPs size distribution in the (x , j ) plane was also extracted from the 2-D Fourier transform of AFM images of NPs using the relation
$$ I\left({k}_x,{k}_y\ \right)=\iint f\left(x,y\right)\exp \left(i{k}_xx\right)\exp \left(i{k}_yy\right) dxdy $$waar f (x , y ) is a size function at a point (x, y ), k x , k j are the associated wavevectors in the inverse Eukledian space at the same point and I (k x , k j ) is the “spectral density” of the function f (x , j ) at the point k x , k j . For most applications, f (x , y ) is the z -height of the NPs at the point (x, y ) and z = f (x , y ).
For a set of discrete data, such as the digitised AFM images, the 2D-FFT was used instead of 2D Fourier transform in the continuous space. For a m × n X-matrix (pixels of an AFM image), the 2D-FFT transform takes the form
$$ \kern1em {Y}_{p+1,q+1}=\sum \limits_{j=0}^{m-1}\sum \limits_{k=0}^{n-1}{\omega}_m^{jp}{\omega}_n^{kq}{X}_{j+1,k+1\kern1.25em } $$where \( {\omega}_m^{jp}={e}^{2 pi/m},{\omega}_n^{kq}={e}^{2 pi/n} \) are the associated frequencies. Then, an appropriate shift along the y -axis was performed and the integers m, n, p, q, k were translated into lengths and inverse lengths respectively by a multiplication with the pixel’s size of the image.
Water Trapping in RE-NPs
VUV Spectroscopy
To appraise the state of water in RE-NP’s complexes during the initial stage of suspension preparation, the adsorption of water molecules on the surface of the hygroscopic PrF3 NPs was identified with a laboratory-made VUV (110–180 nm) absorption spectrometer. It consists of a hydrogen lamp operating in a longitudinal stabilised discharge mode at 10 kV, a stainless steel vacuum chamber and a VUV monochromator (Acton VM502), equipped with a solar blind photomultiplier (Thorn EMI 9412 CsTe) and a laboratory-made data collection system. Thin layers of PrF3 NPs suspensions in water were prepared and dried on 1-mm-thick VUV-grade CaF2 substrates by applying the “drop-casting method”. Then, the CaF2 substrates were placed in the optical path between the hydrogen lamp and the VUV monochromator in a vacuum. The stainless steel 316 vacuum chamber was evacuated initially to 10 − 7 mbar using two turbomolecular pumps at a differential pumping configuration (Edwards EXT 100/200, pumping speed 150 ls −1 ). However, a high outgassing rate of PrF3 sets an upper limit to the background pressure in the vacuum chamber ~ 8.5 × 10 −5 mbar. The relatively low background pressure of both compounds irreversibly damages the VUV optics and the turbomolecular pump after few hours of operation and therefore it sets certain experimental constraints, preventing an equivalent registration of LaF3 spectrum because of high outgassing rates and a low background operating pressure (< 10 −4 mbar). The experimental data (light transmitted through the sample film on CaF2 window) were fitted to a logarithmic response for calculating the transmittance.
Cell Culture and Growth Assay
Cell Growth
The A549 and SW837 cell lines were maintained in DMEM+FBS, whereas the MCF7 lines were in RPMI+FBS. Both media supplemented with 10% fetal bovine serum (FBS), 1 × penicillin, 1 × streptomycin and 2 mM l-glutamine. Cells were incubated at 37 °C, 5% CO2 in a humidified atmosphere.
The WST viability test was used to monitor the intrinsic toxicity of PrF3 and LaF3 NPs for three human cancer cell lines, A549, SW837 and MCF7. For the viability assay, three different concentrations of RE solubles (0.5, 1 and 5 mM) in DMEM+FBS (A549, SW837) and RPMI+FBS (MCF7) were used. The initial number of cells seeded in the 96-well plates was ~ 5 × 10 4 cellen/put. This amount of cells was plated 24 h prior to the RE-NPs treatment of cells in order to allow enough time for the cells to attach properly to the plate (wells) and to attain the optimum growing conditions. Subsequently, the viability test was performed 24 h after RE-NPs addition, or 48 h after the initial cell cultures were placed in the wells. As we did not observe any cell reduction, but on the contrary cell-overgrowth, especially with the SW620 cell line at 5 mM, the cell confluence quickly reached 80–90% of its initial value after 24 h of the addition of RE-NPs or 48 h from the initial plating.
Five microliters of WST solution was added to each well and the plate was incubated for 1 h during the growth state. The absorbance at 450 nm of each well was measured using a microplate reader (Biorad, x Mark). Each experimental point for each cell line and each RE suspension was extracted from two samples and triplicated every 2 days (total of 108 samples).
F test was used for every set of cell viability measurements. Here, the “null hypothesis” was that the relative to the CTRL “mean viability value was the same at different concentrations within the same cell line”. With this null hypothesis, an unknown law connecting tumour cell viability and RE-NPs concentration was identified. De p value (probability the null hypothesis to be rejected) was also tested from the F distribution Additional file 1.
Western Blotting and Antibodies
Total proteins were extracted with 60 μL of radioimmunoprecipitation assay (RIPA) lysis buffer (20 mM Tris-HCl (pH 7.5); 150 mM NaCl, 1 mM Na2 EDTA; 1 mM EGTA; 1% NP-40; 1% sodium deoxycholate; 2.5 mM sodium pyrophosphate; supplemented with proteases inhibitors 1 mM β-glycerophosphate; 1 mM Na3 VO4 1 μg/ml; leupeptin) and the Wb assay was performed according to standard protocols (Fig. 9b). Briefly, total proteins (50 μg) were separated by SDS-polyacrylamide gel electrophoresis (SDS-PAGE) and transferred to nitrocellulose membrane. Blots were incubated overnight at 4 °C with appropriate primary antibodies. The antibodies used were tubulin code sc-8035, from Santa Cruz (final concentration 1:1000 in blocking buffer); p-ERK (E-4) code sc-7383, from Santa Cruz (final concentration 1:500 in blocking buffer); and p-AKT (Thr308) code 9275S, from Cell Signaling (final concentration 1:1000 in blocking buffer).
Wb bands are collected from different blots showing quality control of antibodies specificity. Numbers at the top of the phosphorylation images show grey scale levels from 0 (black) to 168 (grey) (maximum value ), indicating activation at a non-saturated mode.
Afkortingen
- 2D-FFT:
-
Two-dimensional fast Fourier transform
- ADMIDAS:
-
Adjacent MIDAS
- AFM:
-
Atoomkrachtmicroscopie
- AKT:
-
Protein kinase B
- CTRL:
-
Control cells
- DLS:
-
Dynamische lichtverstrooiing
- DMEM:
-
Dulbecco's gemodificeerde Eagle's medium
- ECM:
-
Cell-extracellular matrix
- EGFR:
-
Epidermal growth factor receptors
- ERK:
-
Extracellular signal-regulated kinase
- F.A.:
-
Feret angle
- F.D.:
-
Feret area diameters
- FBS:
-
Foetaal runderserum
- LABS:
-
Ligand adhesion binding site
- MEAC:
-
Mean equal area circle
- MHR:
-
Mean hydrodynamic radius
- MIDAS:
-
Metal ion-dependent adhesion sites
- NGFR:
-
Nerve growth factor receptor
- NP:
-
Nanodeeltje
- RE-NPs:
-
Rare-earth nanoparticles
- RIPA:
-
Radioimmunoprecipitation assay
- RMS:
-
Root mean square
- RPMI:
-
Roswell Park Memorial Institute medium
- SDS-PAGE:
-
Sodium dodecyl sulfate-polyacrylamide gel electrophoresis
- SyMBS:
-
Synergistic metal ion binding sites
- TEM:
-
Transmissie-elektronenmicroscopie
- TSR:
-
Transmembrane signal receptors
- VEGFR:
-
Vasculaire endotheliale groeifactor
- VUV:
-
Vacuum ultraviolet
- Wb:
-
Western blot assays
- WST:
-
Water-soluble tetrazolium salts
- XRD:
-
Röntgendiffractie
Nanomaterialen
- Over halfgeleidende nanodeeltjes
- Biocompatibele FePO4-nanodeeltjes:medicijnafgifte, RNA-stabilisatie en functionele activiteit
- In vitro onderzoek naar de invloed van Au-nanodeeltjes op HT29- en SPEV-cellijnen
- Gemodificeerd hypervertakte polyglycerol als dispergeermiddel voor groottecontrole en stabilisatie van gouden nanodeeltjes in koolwaterstoffen
- Bevordering van SH-SY5Y-celgroei door gouden nanodeeltjes gemodificeerd met 6-mercaptopurine en een neuron-penetrerend peptide
- Maghemiet-nanodeeltjes werken als nanozymen en verbeteren de groei en abiotische stresstolerantie in Brassica napus
- Near Infrared-Emitting Cr3+/Eu3+ Co-gedoteerde zinkgallogermanaat Persistentie Luminescente nanodeeltjes voor celbeeldvorming
- Op grafeenoxide gebaseerde nanocomposieten versierd met zilveren nanodeeltjes als antibacterieel middel
- Ronde gouden nanodeeltjes:effect van deeltjesgrootte en concentratie op de wortelgroei van Arabidopsis thaliana
- In situ synthese van bimetaal wolfraam-koper nanodeeltjes via reactief radiofrequent (RF) thermisch plasma
- Poly (γ-glutaminezuur) bevordert verbeterde dechlorering van p-chloorfenol door Fe-Pd-nanodeeltjes