Liposomal Nanomedicine:Applications for Drug Delivery in Cancer Therapy
Abstract
De toenemende prevalentie van kanker, een ziekte waarbij snelle en oncontroleerbare celgroei complicaties en weefseldisfunctie veroorzaakt, is een van de ernstige en gespannen zorgen van wetenschappers en artsen. Tegenwoordig wordt de diagnose van kanker en vooral de effectieve behandeling ervan beschouwd als een van de grootste uitdagingen op het gebied van gezondheid en geneeskunde van de vorige eeuw. Ondanks aanzienlijke vooruitgang in het ontdekken en afleveren van geneesmiddelen, waren hun vele nadelige effecten en ontoereikende specificiteit en gevoeligheid, die gewoonlijk schade aan gezonde weefsels en organen veroorzaken, een grote belemmering bij het gebruik ervan. Beperking van de duur en hoeveelheid van de toediening van deze therapeutische middelen is ook een uitdaging. Aan de andere kant benadrukt de incidentie van tumorcellen die resistent zijn tegen typische methoden van kankerbehandeling, zoals chemotherapie en radiotherapie, de intense behoefte aan innovatie, verbetering en ontwikkeling van de eigenschappen van antitumorgeneesmiddelen. Liposomen zijn gesuggereerd als een geschikte kandidaat voor medicijnafgifte en kankerbehandeling in nanogeneeskunde vanwege hun vermogen om medicijnen met verschillende fysieke en chemische kenmerken op te slaan. Bovendien is de hoge flexibiliteit en het potentieel van de liposoomstructuur voor chemische modificatie door het conjugeren van verschillende polymeren, liganden en moleculen een belangrijk voordeel voor liposomen, niet alleen om hun farmacologische verdiensten te verbeteren, maar ook om de effectiviteit van geneesmiddelen tegen kanker te verbeteren. Liposomen kunnen de gevoeligheid, specificiteit en duurzaamheid van deze anti-maligne celmiddelen in het lichaam verhogen en bieden opmerkelijke voordelen voor toepassing in nanomedicijnen. We hebben de ontdekking en ontwikkeling van liposomen beoordeeld, gericht op hun klinische toepassingen voor de behandeling van verschillende soorten kankers en ziekten. Hoe de eigenschappen van liposomale geneesmiddelen kunnen worden verbeterd en hun kansen en uitdagingen voor kankertherapie werden ook overwogen en besproken.
Grafische samenvatting
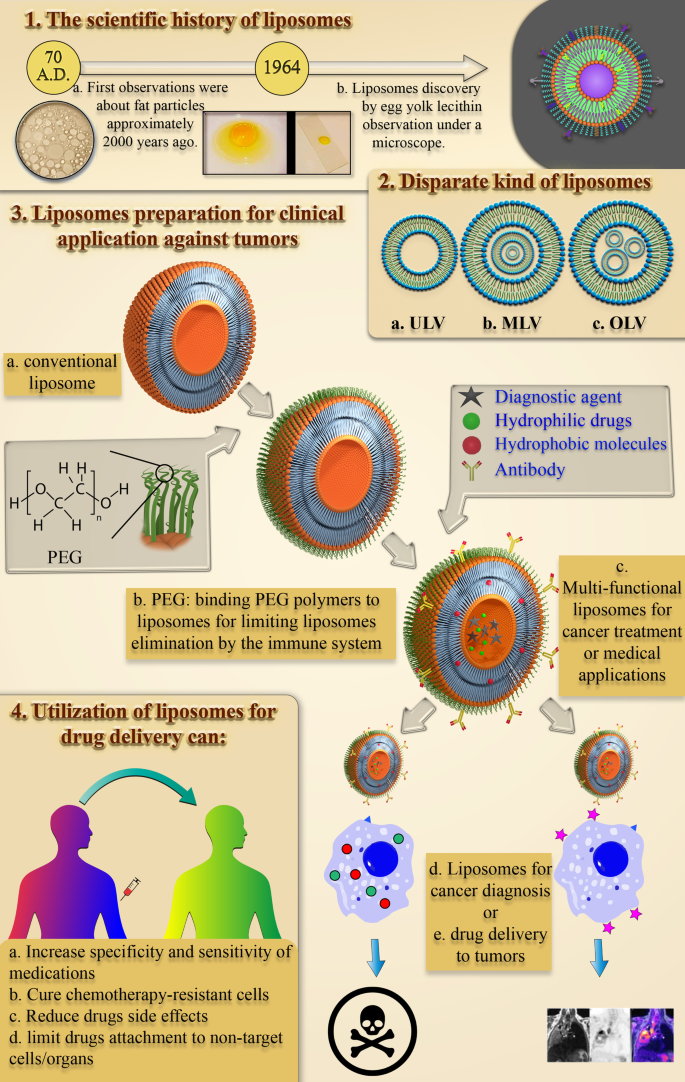
Inleiding
Kanker, een ziekte waarbij de gezonde lichaamscellen uit hun normale toestand raken en zich ongecontroleerd delen, wordt in de huidige eeuw erkend als een grote medische uitdaging. Deze complicatie wordt veroorzaakt door de accumulatie van kankerverwekkende stoffen in de omgeving of genetische mutaties [1] en wordt in de huidige eeuw erkend als een grote medische uitdaging. Miljoenen mensen sterven elk jaar als gevolg van kanker, en het aantal nieuwe patiënten en het sterftecijfer neemt voortdurend toe [2]. Volgens rapporten van de Wereldgezondheidsorganisatie (WHO) was kanker de tweede belangrijkste doodsoorzaak in 2018, en een schatting toonde aan dat in dat jaar ongeveer 9,6 miljoen mensen stierven aan verschillende vormen van kanker. In 2018 werd ongeveer 1 op de 6 sterfgevallen veroorzaakt door kanker. Ongeveer 70% van de sterfgevallen door kanker vindt plaats in ontwikkelingslanden en lage-inkomenslanden. Er moet echter ook rekening worden gehouden met de incidentie en het sterftecijfer van kanker in ontwikkelde landen [3].
Chemotherapie met antitumormiddelen staat bekend als een belangrijke behandeling van kanker [4]. Chemotherapie met gratis medicijnen is beperkt vanwege een gebrek aan geschikte sensitiviteit en specificiteit. Als gevolg hiervan heeft deze beperking een nauwkeurige behandeling verhinderd vanwege bijwerkingen en voldoende inspanning van het antitumoreffect geremd [5]. Chemo-immunotherapie, een gelijktijdige gecombineerde behandeling, is ook gesuggereerd als een effectieve en veelbelovende methode voor kankertherapie, waarbij expliciet tumorcellen worden behandeld die resistent zijn tegen conventionele medicijnen. In de afgelopen jaren is een verscheidenheid aan conventionele en geavanceerde behandelingen ontdekt en toegepast om kanker te behandelen. Om bijvoorbeeld de bijwerkingen van conventionele geneesmiddelen tegen kanker te verminderen, met name chemotherapie, verschillende nanomedicijnen [6], waaronder virale nanodeeltjes (VNP's) [7, 8], kwantumdots [9], polymere nanomaterialen [10] en liposomen [11] zijn toegepast.
Van de verschillende nanomedicijnen hebben liposomen als sferische nanodeeltjes (NP's) een bepaalde structuur. De aanwezigheid van twee waterige en organische fasen in het liposoombestanddeel maakt het invangen van beide soorten hydrofiele en hydrofobe middelen mogelijk en creëert een opmerkelijk voordeel voor het liposoom ten opzichte van veel nanodragers. Een van de manieren om de specificiteit, biologische beschikbaarheid en biocompatibiliteitseigenschappen van antitumormiddelen te verbeteren, is door ze in verschillende soorten liposomen op te sluiten [5]. In de afgelopen twee decennia zijn er aanzienlijke inspanningen gedaan om liposomen voor therapeutische doeleinden te exploiteren. Sommige van deze geneesmiddelen, zoals DaunoXome® en Caelyx®, zijn goedgekeurd voor algemene en klinische toepassingen, terwijl andere zich in de laatste productie- en goedkeuringsfase bevinden [12].
Over het algemeen zijn er verschillende soorten therapeutische liposomen, zoals immunoliposomen en pH-gevoelige liposomen. Immunoliposomen zijn een grote groep nanomedische apparaten, ook bekend als gerichte medicijnafgiftesystemen (DDS's), die significante anti-maligne effecten hebben laten zien in studies en onderzoeken [13]. pH-gevoelige liposomen zijn ook bekend als een groep polymorfe liposomen, waarbij de structuur en samenstellende moleculen worden veranderd door pH-verandering, wat leidt tot het vrijkomen van hun geneesmiddelgehalte [14]. Bovendien hebben liposomen, net als andere nanomedicijnen, het potentieel om te worden gebruikt voor weefselherstel en -regeneratie, beeldvorming en diagnose, naast systemen voor medicijnafgifte. Het gebruik van liposomen in verschillende aspecten vereenvoudigt de identificatie, het beheer en de behandeling van ziekten en kankers [15].
In dit artikel wordt een samenvatting gegeven van de bevindingen over de ontdekking en structuur van liposomen, verschillende eigenschappen van liposomen en liposomale geneesmiddelen voor de behandeling van kanker die op de markt zijn en hun ontwikkeling. Uiteindelijk zal een rapport worden opgesteld over de kansen en uitdagingen van het gebruik van liposomale nanomedicijnen, wat kan worden benadrukt als cruciale kwesties die moeten worden opgemerkt in toekomstig onderzoek van wetenschappers, wat zal leiden tot het wegnemen van de beperkingen en het versterken van de positieve punten.
Hoofdtekst
De wetenschappelijke geschiedenis van liposomen:ontdekking en definitie
Het duurde ongeveer 1950 jaar van vroege studies naar de structuur en het gedrag van kleine lipidedeeltjes in een waterige omgeving tot het eerste door de Amerikaanse FDA goedgekeurde op lipiden gebaseerde nanodeeltje voor medicijnafgifte. Het proces van het bestuderen van het gedrag van lipiden en vetdeeltjes in aqua begon met de eerste waarnemingen door Plinius de Oudere, bijna 2000 jaar geleden [16]. Aan het eind van de zeventiende eeuw riep de ontdekking van de cel door Anthonie Van Hook veel vragen op over de structuur van cellen [17]. Toen ontdekten Gorter en Grendel de aanwezigheid van fosfolipide dubbellagen in celmembranen [18]. Vervolgens beschreven Singer en Nicolson later het dubbellaagse mozaïekmembraanmodel om het gedrag van celmembraanfosfolipiden te verklaren [19]. Deze wetenschappelijke observaties en hypothesen trokken de aandacht van de andere wetenschappers naar van vet afgeleide NP's. In de jaren zestig observeerde Alec D. Bangham, die het effect van lipiden, met name fosfolipiden, op het bloedstollingsproces aan het Babraham Institute [20, 21] bestudeerde, per ongeluk de eerste liposomen en was verrast om spontane bolvormige deeltjes te zien vormen in de water [22]. Daarna noemde Gerald Weissmann, een bezoeker van het laboratorium van Alec Bangham die op de hoogte was van de resultaten van het onderzoek van Bangham, de door Alec waargenomen Smectic Mesophase "liposomen" in plaats van "banghosomes" en ontving hij de Nobelprijs [22]. De wetenschappelijke geschiedenis van de ontdekking van liposomen is samengevat in figuur 1.
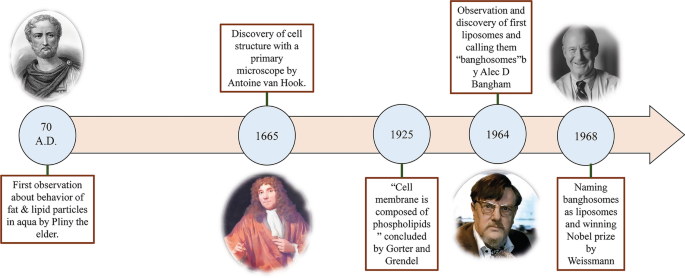
Schema van waarnemingen die hebben geleid tot de ontdekking van liposomen. De historische en wetenschappelijke trends van het bestuderen van het gedrag van lipide- en vetdeeltjes in water en de waarnemingen die hebben geleid tot de ontdekking van liposomen, samen met beelden van de wetenschappers die bij het evenement betrokken waren, Plinius de Oudere [23], Anthonie Van Hook [24], Alec D. Bangham [25] en Gerald Weissmann [26], respectievelijk, van links naar rechts
Welke structuren staan tegenwoordig bekend als liposomale nanodeeltjes?
Er is een intense poging om liposomale NP's te definiëren en hun eigenschappen redelijk te ontdekken. Tegenwoordig worden liposomen gedefinieerd als spontaan vormende en bolvormige fragmenten die bestaan uit een dubbellaags membraan van lipiden en een hydrofiele kern.
Liposomen variëren in grootte van ongeveer 10 nm tot 2500 nm (of 2,5 µm) [15]. De meeste liposomen die voor medicijnafgifte worden toegediend, zijn echter typisch ongeveer 50 tot 450 nm groot. Absoluut, liposomen met veel grotere afmetingen kunnen ook worden gebruikt voor medische toepassingen [27]. Verder zijn liposomen voornamelijk samengesteld uit fosfolipiden. Fosfolipiden zijn een soort lipiden, die interessant lijken op triglyceriden. In de structuur van fosfolipiden is er een hydrofiele pool en twee hydrofobe ketens. Fosfolipiden worden dus beschouwd als amfifiele moleculen.
Het liposoommembraan van fosfolipiden omvat meestal fosfatidylcholine (PC), sfingomyeline (SM), fosfatidylserine (PS) en fosfatidylethanolamine (PE), die amfifiel zijn en een sterke neiging hebben om bepaalde structuren in water te vormen [28]. De fysieke reden voor dit fenomeen is de gelijktijdige aanwezigheid van een hydrofiele kop (fosfaatmolecuul) en twee hydrofobe staarten (vetzuren) in fosfolipiden. De fosfaatgroep interageert met H2 O polaire moleculen, terwijl de hydrofobe staarten ontsnappen aan watermoleculen en met elkaar interageren [29]. In dit geval worden de niet-polaire ketens tegenover elkaar geplaatst en vormen ze een dubbellaag, waardoor er een lipofiele ruimte tussen hen ontstaat. Dienovereenkomstig kan deze lipofiele deelstructuur van de liposomen worden toegepast om hydrofobe middelen en materialen op te slaan. Bovendien wordt het hydrofiele deel van het fosfolipide vervolgens naar de watermoleculen geleid via moleculaire krachten, zoals waterstofbruggen, Van der Waals, enz., Die daartussen verschijnen. Deze leiden tot de vorming van een hydrofiel gebied in de liposomen. De structuur van het lecithinemolecuul, als een natuurlijk fosfolipide dat overvloedig aanwezig is in eigeel en in staat is om liposomen te vormen in water en verschillende delen van een liposoom, wordt getoond in Fig. 2.
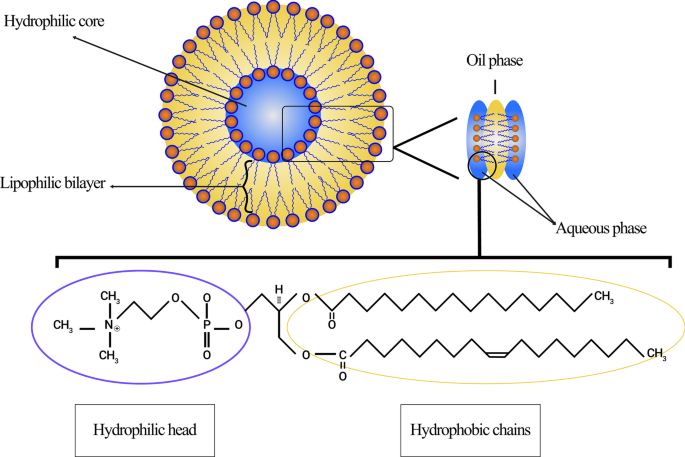
Schematische figuur van liposomen afkomstig van lecithine. Verschillende regio's van liposomen, waaronder hydrofiele kern en hydrofobe dubbellaag, worden gedemonstreerd. De structuur van het lecithinemolecuul, zijn hydrofiele pool en hydrofobe ketens zijn gespecificeerd
Ook de bolvormige structuur van liposomen na oplossen in water, afhankelijk van het type moleculen, temperatuur van het waterige medium, molaire concentratie en de aanwezigheid van andere stoffen, zoals ionen, bepaalt de uiteindelijke vorm [30]. De overheersende fysieke en chemische eigenschappen van een liposoom zijn de netto-eigenschappen van de samenstellende lipiden, vooral fosfolipiden en de andere moleculen waaruit het bestaat. Deze eigenschappen omvatten permeabiliteit, oppervlakteladingsdichtheid en totale grootte [31].
Verschillende typen liposomenclassificatie
Sinds de ontdekking van liposomen zijn deze structuren altijd gebruikt als een essentieel onderdeel van biologisch, biofysisch, biochemisch of farmaceutisch onderzoek.
Tegenwoordig kunnen liposomen worden gecategoriseerd op basis van hun grootte, het aantal van hun fosfolipidedubbellaag, syntheseprocedure en bereidingsmechanisme. Qua grootte kunnen liposomen in drie groepen worden verdeeld:klein, middelgroot en groot. Gezien het aantal membraanlagen, kunnen dit unilamellaire blaasjes (ULV's), oligolamellaire blaasjes (OLV's) en multilamellaire blaasjes (MLV's) zijn. In dit opzicht zijn ULV's liposomen die zijn samengesteld uit één fosfolipidedubbellaag van ongeveer 50 tot 250 nm, terwijl MLV's veel groter zijn, ongeveer 0,5-1,5 µm, en verschillende fosfolipidedubbellaagmembranen bevatten [32]. Verschillende synthesemethoden veroorzaken marges tussen deze twee groepen. In termen van toepassing hebben ULV's ook intern een grote hydrofiele omgeving, waardoor ze geschikt zijn voor het invangen van hydrofiele geneesmiddelen. Kleine unilamellaire blaasjes (SUV's), zoals ULV's, zijn samengesteld uit één fosfolipide dubbellaag, maar qua afmeting zijn ze minder dan 100 nm groot [33, 34]. Vanuit morfologisch oogpunt zijn OLV's liposomen die zijn samengesteld uit twee tot vijf blaasjes die identieke of verschillende groottes kunnen hebben. In de structuur van OLV's zijn de blaasjes allemaal ingesloten in één grote fosfolipide dubbellaag zonder in elkaar te zitten. OLV's zijn meestal ongeveer 0,1-1 µm [33,34,35]. In tegenstelling tot ULV's zijn MLV's niet ideaal voor de afgifte van hydrofiele stoffen. MLV's worden meestal gebruikt voor de afgifte van hydrofobe middelen [36]. Verschillende soorten liposomen worden geïllustreerd in figuur 3.
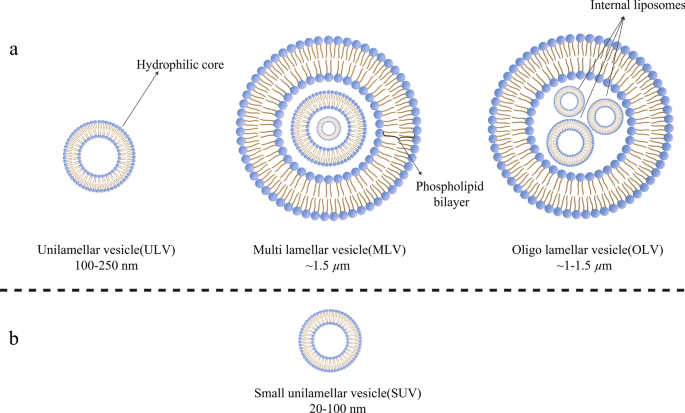
Classificatie van liposomen volgens verschillende criteria:a Liposomen zijn qua grootte onderverdeeld in drie categorieën; b De structuur van de kleine unilamellaire blaasjes (SUV), als lid van de unilamellaire blaasjes (ULV's), die een opvallend kleine omvang heeft
Voorbereidingsmethoden voor liposomen en ontwikkeling van hun nieuwe generaties
In tegenstelling tot gouden nanodeeltjes als harde NP's, zijn liposomen zachte NP's [37] en kunnen ze via verschillende methoden worden gesynthetiseerd. MLV's en ULV's hebben bijvoorbeeld verschillende bereidingsmechanismen. Bij de meeste van deze methoden wordt een bepaald oplosmiddel (zoals chloroform of methanol, enz.) gebruikt om lipiden op te lossen die bedoeld zijn om liposoommembranen te vormen (met de gewenste molaire verhouding) in een rondbodemkolf (RBF). Handen schudden is bijvoorbeeld de primaire procedure om MLV's te synthetiseren [38]. Tijdens deze procedure, die ook wordt herkend als lipidefilmhydratatie, worden lipiden toegevoegd aan een organisch oplosmiddel. Vervolgens wordt het oplosmiddel verdampt door een roterende inrichting en wordt het vaste product gelipolyseerd. Uiteindelijk worden liposomen gesynthetiseerd volgens hydratatie- en extrusiemethoden [39]. Andere methoden voor liposoomsynthese omvatten ultrasoonapparaat, omgekeerde faseverdamping, Franse drukcel, vriesdrogen en membraanextrusie [38, 40].
Bovendien kunnen liposomen ook in verschillende categorieën worden besteld op basis van hun ontdekking en ontwikkeling in de loop van de tijd. Liposomen van de eerste generatie worden in het algemeen conventionele of klassieke liposomen genoemd. Problemen die werden waargenomen bij het gebruik van conventionele liposomen als therapeutische NP's, werden zo snel in vivo geïdentificeerd. Een vroeg onderzocht probleem was de beperking van het opsluiten van geneesmiddelen in liposomen. Met andere woorden, veel medicijnen konden niet worden opgeslagen in liposomen van de eerste generatie [41]. Samen met de grote wens om de structuur en eigenschappen van liposomen te onderzoeken, zoals stabiliteit, therapeutische werkzaamheid en de mogelijkheid van klinische toepassingen, leidden deze uitdagingen tot de ontwikkeling van liposomen van de tweede generatie door de samenstellende lipiden, oppervlaktelading, nettogewicht, en totaal volume [42]. Om precies te zijn, liposomen van de tweede generatie worden voornamelijk gesynthetiseerd door enkele hydrofiele polymeren toe te voegen aan conventionele liposomen voor de verbetering van hun houdbaarheid in lichaamsvloeistoffen om ze geschikte kandidaten te maken voor medicijnafgiftesystemen. Dit soort liposoom kan in twee groepen worden verdeeld:niet-specifieke lang-circulerende liposomen of ligand-gerichte lang-circulerende liposomen [43].
Archeosomen, als een nieuwe generatie liposomen, bestaan uit het archaeale membraanlipide en synthetische fosfolipidenanalogen. In het afgelopen decennium zijn uitgebreide en aanzienlijke inspanningen geleverd om het potentieel van archeosomen te onderzoeken voor hun toepassing bij de toediening van geneesmiddelen en vaccins. De structurele kernen van lipiden van het archaea-type zijn di-ether- of tetra-ethermoleculen met verzadigde fytanylketens die ongeveer 20 tot 40 koolstofatomen bevatten. Deze koolstofketens hechten aan de etherbindingen van sn-2,3-koolstoffen van de ruggengraat glycerol die wordt aangetroffen in archaeol of caldarchaeol. Zoals hierboven vermeld, kunnen deze deeltjes ook enorm worden gebruikt bij de toediening van medicijnen voor neoplastische complicaties, allergieën en infecties, evenals vaccinaties [44].
Evaluatie van de biomateriaalkenmerken en fysisch-chemische eigenschappen van liposomen
Zoals eerder vermeld, staat de behandeling van sommige ziekten, met name kanker, ondanks de wijdverbreide vooruitgang in de medische wetenschappen nog steeds voor enorme uitdagingen als gevolg van de inefficiënte therapeutische middelen en methoden. Het aanpassen van de geïnjecteerde medicijndosis om tumoren te beïnvloeden is een gespannen kwestie vanwege het smalle therapeutische venster van antikankermiddelen. Met andere woorden, een kleine afstand tussen de therapeutische en de toxische dosis, evenals ongepaste gevoeligheid en specificiteit, hebben geleid tot een grote vraag naar geavanceerde remediërende procedures [42].
Bovendien heeft het gebruik van nanomaterialen voor de afgifte van geneesmiddelen aan weefsels de laatste tijd de aandacht getrokken. Biocompatibiliteit en biologische afbreekbaarheid zijn twee essentiële kenmerken van biomateriaalkenmerken voor het gebruik van nanomaterialen in afgiftesystemen. Biocompatibiliteit is vereist om te voorkomen dat therapeutische NP's de lichaamsweefsels en -systemen beschadigen, en biologische afbreekbaarheid is dringend noodzakelijk om NP's af te breken in niet-toxische verbindingen en ze eenvoudig uit de organen te verwijderen [15]. Na detectie van liposomen begonnen wetenschappers ze toe te passen als nanomaterialen voor medicijnafgifte. Zoals vermeld, hebben liposomen twee vereiste biomateriaaleigenschappen voor therapeutische doeleinden:biocompatibiliteit en biologische afbreekbaarheid [36]. Ook hebben liposomale NP's andere eigenschappen die ze geschikt maken voor dit doel. Vanwege de specifieke structuur van liposomen kunnen bijvoorbeeld beide groepen hydrofiele (in water oplosbare) en hydrofobe (in vet oplosbare) geneesmiddelen erin worden ingekapseld. Bovendien beschermt de aanwezigheid van een fosfolipide dubbellaags membraan in liposomen de middelen die in liposomen zijn opgeslagen tegen verschillende verschijnselen en beschadigingen, zoals enzymdegradatie, biologische inactivatie door immunologische structuren en chemische veranderingen in vivo. Dit punt heeft twee belangrijke voordelen:ten eerste blijft de structuur van de moleculen die in het liposoom zitten, behouden voordat ze het doelweefsel bereiken, en worden er geen wijzigingen in aangebracht, en ten tweede worden andere gezonde en niet-doelweefsels beschermd tegen blootstelling aan de geneesmiddel vanwege het liposoommembraan en kan niet worden beïnvloed door deze middelen [42]. Liposomen kunnen ook worden toegepast voor het afleveren van genetisch materiaal, zoals DNA, RNA, enz. en voor gentherapiedoeleinden. Liposomen die voor dit doel worden gebruikt, kunnen zijn samengesteld uit kationische, anionische, neutrale lipiden en fosfolipiden of een mengsel daarvan [45]. Sommige diagnostische en beeldvormende middelen, zoals koolstofstippen, kunnen worden gebruikt voor kankerdetectie en beeldvorming in de combinatie van liposomen of afzonderlijk [46]. Hoewel koolstofstippen gedeeltelijk zijn goedgekeurd voor klinische toepassing en worden gebruikt in onderzoeken, blijft cytotoxiciteit een uitdagende barrière voor hun brede toepassing [47]. De algemene structuur van liposomale geneesmiddel-NP's wordt getoond in figuur 4.
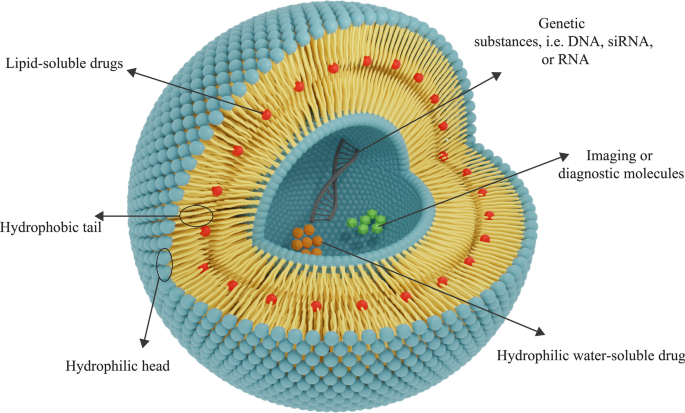
De algemene structuur van de liposomen bestaat uit fosfolipidelagen. Afhankelijk van de hydrofiliciteit-hydrofobiciteit van een medicijn, zal het juiste soort liposomen voor de afgifte worden bepaald. Hydrofiele geneesmiddelen worden ingesloten in de centrale hydrofiele kern en hydrofobe geneesmiddelen worden in het lipofiele gebied geplaatst. Liposomen kunnen ook worden gebruikt voor de levering van genen
De membraanvormende fosfolipiden die in liposomen aanwezig zijn, zijn niet-toxische verbindingen en kunnen worden gesynthetiseerd in een uitgebreide reeks groottes. De fysisch-chemische eigenschappen van liposomen zijn afhankelijk van hun bestanddelen. Zo kunnen liposomen met de gewenste eigenschappen worden gesynthetiseerd door bepaalde verbindingen toe te voegen, zoals cholesterol, polyethyleenglycol (PEG), enz. Bovendien is het membraan van liposomen ondoordringbaar voor gigantische moleculen, wat helpt om materiaal beter in het liposoom vast te houden [48] ]. Alle genoemde kenmerken van het liposoom introduceren het als een geschikt nanomateriaal voor gebruik bij de levering van therapeutische middelen voor de behandeling van verschillende ziekten, met name kanker.
Gregory Gregoriadis, als een van de pioniersonderzoekers op dit gebied, stelde de hypothese voor om liposomen te gebruiken voor de medicijnafgiftesystemen en stelde dat geneesmiddelverbindingen in liposomen kunnen worden opgesloten [49]. De juiste biomateriaal en fysiochemische eigenschappen van liposomen zijn gerapporteerd. Zo heeft een onderzoek naar liposomen die het antitumormiddel cytosine arabinoside bevatten dat in diermodellen wordt gebruikt, een significante toename van de levensduur van muizen met L1210-leukemie aangetoond [50]. Door liposomen toe te passen, kan een voldoende dosis van de actieve vorm van het medicijn op een beschermde manier op de doelplaats worden afgeleverd [42].
Verhoging van specificiteit en gevoeligheid van liposomale NP's voor therapeutische toepassingen
Zoals eerder vermeld, maakt het gebruik van verschillende moleculen en polymeren het mogelijk om de structuur en het membraan van liposomen te veranderen, en hierdoor kunnen nieuwe functionaliteiten aan liposomen worden toegevoegd of hun eigenschappen worden gewijzigd [51]. Zowel het verlengen van de circulatie van liposomen in het bloed als het vergroten van hun vermogen om zich op te hopen in specifiek tumorweefsel of pathologische plaats via het EPR-effect zijn de eerste belangrijke kenmerken waarmee rekening moet worden gehouden vanwege de hoge mate van klaring van liposomen. De conjugatie van PEG-moleculen aan liposoommembranen door chemische conjugatie is achtereenvolgens gebruikt om deze functionaliteit aan liposomen toe te voegen [52]. Het belang en de rol van ethyleenglycolpolymeren bij het verlengen van de halfwaardetijd van liposomen, met name de liposomale therapeutische NP's in lichaamsvloeistoffen, zoals bloed, werden ongeveer 20 jaar geleden tot uitdrukking gebracht [53].
Abuchowski en McCoy deden de eerste pogingen om de halfwaardetijd van liposomen in de bloedbaan te verlengen door PEG aan hun structuur te conjugeren. Als gevolg hiervan verhoogden hun inspanningen in het algemeen de circulatietijd van liposomen en hun halfwaardetijd in de bloedbaan [54]. Na een paar maanden onderzochten andere onderzoekers de mogelijkheid om de hoge snelheid van klaring van liposomen door mononucleaire fagocytose-systeemcellen (MPS) te verminderen. Door PEG te hechten aan de oppervlaktemoleculen van de liposomen [53], wordt verwacht dat de liposomencirculatie in het bloed verbetert. Er is een veelheid aan artikelen op dit gebied. Bovendien vertoonden PEG-gecoate liposomen, in tegenstelling tot conventionele liposomen, dosisonafhankelijke farmacodynamische eigenschappen [55]. Van de verschillende polymeren zijn PEG-moleculen een van de polymeren die aan het liposoomoppervlak kunnen worden gehecht om hun houdbaarheid in vivo te verlengen. Hiervoor kunnen ook andere polymeren worden gebruikt [56]. Afbeelding 5 laat zien hoe de PEG-polymeermoleculen het liposoom kunnen beschermen tegen antilichamen en ook de levensduur in de bloedbaan kunnen verlengen.
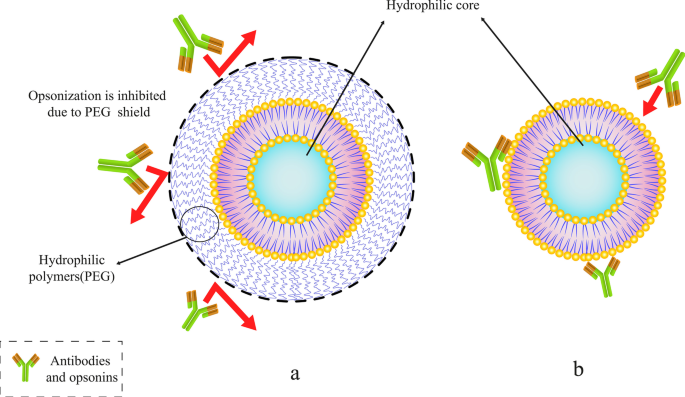
Conjugeren van een specifiek polymeer zoals polyethyleenglycol (PEG) aan liposomen. een PEGylated liposomen met PEG polymeer moleculen schild. b Conventioneel liposoom gevangen door antilichamen en opsoninen
Zoals vermeld, is het opmerkelijk dat naast PEG ook andere moleculen kunnen worden gebruikt om de circulatie van liposomen te verlengen. Bovendien behoren polyoxazolinenpolymeren tot de stoffen die worden gebruikt om het liposomenmembraan te modificeren om hun halfwaardetijd te verbeteren. In dit verband hebben Woodle et al. waren de eerste groep die poly[2-ethyl-2-oxazoline] (PEOZ) toepaste om stealth-liposomen te synthetiseren. Hun resultaten getuigden van een vermindering van de eliminatie en opname van poly[2-ethyl-2-oxazolylated] PETOXylated-liposomen die in ratten werden geïnjecteerd door lever-miltcellen [57]. Hun resultaten gaven aan dat de conjugatie van andere polymeren zoals poly[2-ethyl-2-oxazoline] en poly[2-mthyl-2-oxazoline] (PMOZ) PEG-achtige effecten zou kunnen hebben bij het verhogen van de halfwaardetijd en het verlengen van de circulatie van liposomen in vivo. Ze vergeleken ook de biodistributie van PEG-, PEOZ- en PMOZ-geconjugeerde liposomen in verschillende organen en systemen. De gevolgen toonden ook aan dat de biodistributie van al deze liposomen in bloed en milt bijna hetzelfde was, maar in de lever was de distributie van PMOZ veel lager dan de andere [57].
Pijn et al. gebonden dextran moleculen aan het oppervlak van ULVs. Hun resultaten toonden aan dat met dextran geconjugeerde liposomen, in vergelijking met conventionele liposomen, een meer uitgebreide circulatie hadden en een lagere absorptie en opname door de lever en milt. Dit gevolg getuigde dat dextraanmoleculen, naast het verlengen van de houdbaarheid van liposomen in het lichaam, ook kunnen worden toegepast voor het verhogen van de stabiliteit en het reguleren van de snelheid van geneesmiddelafgifte uit liposomen [58].
Het tweede punt dat moet worden opgemerkt, is de vloeibaarheid en stabiliteit van liposomen. Andere lipiden, waaronder cholesterol, kunnen worden gebruikt in het kader van liposomen. Cholesterol kan af en toe worden vervangen door sommige verbindingen in de fosfolipidedubbellaag om bepaalde eigenschappen van het liposoom te verbeteren. Desalniettemin is bewezen dat het wijzigen van de inhoud van de liposoomdubbellaag en het vervangen van sommige van de fosfolipidemoleculen door bepaalde verbindingen, met name cholesterol, de vloeibaarheid van liposomen kan verminderen [59]. Bovendien verhoogt de aanwezigheid van cholesterol in het membraan van liposomen de stabiliteit van hun structuur (zowel in vivo als in vitro experimenten). Het vermindert ook de doorlaatbaarheid en de kans op lekkage van ingesloten stoffen. Cholesterol is een hydrofobe steroïde die interageert met de hydrofobe ketens tussen fosfolipide dubbellagen om de structuur ervan te stabiliseren wanneer aanwezig in het membraan van liposomen. Deze werking van cholesterol is aanzienlijk wanneer liposomen klinisch in vivo worden gebruikt, omdat het voorkomt dat het liposoom in het lichaam wordt omgezet in lipoproteïne met hoge dichtheid (HDL) en lipoproteïne met lage dichtheid (LDL). Bovendien kunnen in het bloed en intracellulaire vloeistoffen aanwezige lipidestructuren een indruk op liposomen hebben. Lipoproteïnen zoals LDL en HDL beïnvloeden geïnjecteerde liposomen en veroorzaken lipideoverdracht en herschikking van hun membranen. Ze verminderen ook drastisch de stabiliteit van geneesmiddelbevattende liposomale NP's [12]. Het valt op dat andere materialen, zoals DNA en andere moleculen die in liposoommembraan worden gebruikt voor therapeutische toepassingen, aan het cholesterol in het membraan moeten worden verankerd. Het toevoegen van verschillende stoffen aan liposoommembranen is een manier om positieve eigenschappen in liposomen te creëren [42].
De derde essentiële kenmerken waarmee rekening moet worden gehouden, zijn de gevoeligheid en specificiteit van liposomen voor nauwkeurige identificatie en specifieke binding aan doelcellen. Door verbindingen te binden, zoals monoklonale antilichamen, Fab fragmenten en andere conjugatieve moleculen zoals transferrine en folaat, is het mogelijk om de specificiteit van liposomen te versterken, wat resulteert in specifieke binding aan tumorcellen [60]. Bovendien is eerder onderzoek gedaan naar de verbetering van de specificiteit en gevoeligheid van nanodragers van geneesmiddelen, met name liposomen. Mohammad J. Akbar et al. bestudeerde peptide-PEG-lipide-geconjugeerde liposomen voor de behandeling van kleincellige longkanker (SCLC). Hun resultaten toonden aan dat het binden van het Gastrin Releasing Peptide Receptor (GRPR)-antagonistpeptide aan liposomen de specificiteit en accumulatie van deze liposomen in cellen die GRPR tot expressie brengen, zou kunnen verhogen. Ze beweerden ook dat deze aan peptiden gehechte liposomen kunnen worden toegepast om longkankercellen te behandelen vanwege de opregulatie van genen die GRPR tot expressie brengen [61].
Uiteindelijk werden medicijnen en medicijnen toegevoegd aan gePEGyleerde liposomen vanwege hun geschikte eigenschappen, en nu zijn deze liposomale structuren vastgesteld voor industrieel-klinisch gebruik [62]. Antilichamen werden ook in vroege onderzoeken gebruikt om het vermogen van liposomen om aan doelcellen te binden te vergroten [63]. In dit geval werd receptor-gemedieerde endocytose uitgevoerd door liposomen om de cel binnen te gaan [64]. Inmiddels zijn er verschillende methoden ontwikkeld om antilichamen aan liposomen te binden [65]. Studies naar de met antilichamen geconjugeerde liposomen hebben aangetoond dat de toxiciteit van geneesmiddelen tegen kanker tegen gekweekte tumorcellen toeneemt met de conjugatie van antilichamen aan het oppervlak van liposomen [66]. Toen antilichamen op het oppervlak van PEG-liposomen werden aangebracht, werd het overblijfsel van antilichamen om aan hun doelreceptoren te hechten versluierd door PEG-polymeren, vooral wanneer de zijketens die aan de PEG waren gehecht lang waren [67]. Daarom moeten wetenschappers het gelijktijdige gebruik van PEG en antilichaam voor liposomenmedicatie en de nadelen ervan overwegen.
De vierde belangrijke factor bij de therapeutische toepassing van liposomen is de etude van de afgifte van medicijnen die erin gevangen zitten. Aanpassing van liposomen voor de bevrijding van geneesmiddelen erin die zijn aangetast door de abnormale toestand van de beschadigde weefsels is een van de cruciale kwesties bij het klinisch toedienen van liposomen. Bovendien is het gebruik van temperatuurgevoelige verbindingen, pH of een specifieke metaboliet in het oppervlak van liposomen die aan het weefsel- en membraanoppervlak van de doelcellen kunnen binden, een methode om deze medicijnen precies af te geven. Utilizing this method can result in the specific effect of liposomes on the membrane surface of target cells and also release the drug content inside them [68].
The releasing rapidity of compounds entrapped in liposomal NPs is the fifth substantial criterion for adjusting the dose of drugs available at the target site. One of the essential objects that should be considered for the proper usage of all kinds of drug delivery systems, including liposomes, is the releasing rate of drugs and regulation. With regard to liposomal drug delivery systems and NPs, it is worth mentioning that the encapsulated substances in the liposomes are not biologically available and can only be bioavailable while it is released from the initial state. Therefore, drug-containing liposomes can provide the ability to increase the concentration of bioavailable drugs for cancerous tissues and to improve the quality of treatment and therapeutic efficacy can be achieved on condition that the rate of drug release from the liposome is adjusted [69]. Furthermore, it has been proven that changing the liposome bilayer content and replacing some phospholipids with certain compounds, especially steroid molecules like cholesterol, can decrease the permeability and unintended leakage of the compounds stored in them [70]. Consequently, this advantage can be exploited to adjust the release rate of the encapsulated compound. Once released, the drugs must penetrate sufficiently into the cell and make the necessary physiological-biochemical changes to exert their impact.
As it is mentioned earlier, various compounds, including aptamers, can be conjugated to liposomes. In this regard, Mohammad Mashreghi et al. applied anti-epithelial cell adhesion molecule (anti-EpCAM) as an aptamer to functionalize Caelyx® liposomes. Their experiment outcomes determined that functionalization of Caelyx® with this aptamer could enhance the merits of this liposomal drug and made it a viable option for cancer treatment [71]. Figure 6 shows the structure of different types of liposomes that are used in vitro or for clinically scientific purposes schematically.
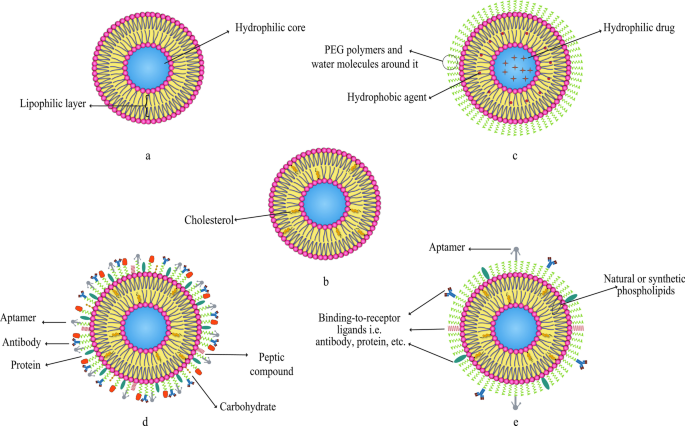
Various kinds of liposomes. een Conventional liposome; b cholesterol-conjugated liposome; c PEGylated or stealth liposome; d ligand-targeted liposome; e multi-functional liposome
The passage of drugs through lysosomes to enter cells (which have low pH and many degrading enzymes) is the sixth most important factor for the practical application of conjugated medicines to liposomes. To protect therapeutic agents from unwanted conversions in extracellular and intracellular space, cell-penetrating peptides are attached to the liposome surface [72].
On Liposomal Drugs Pharmacology:Pharmacokinetics and Pharmacodynamics
The assessment of pharmacological attributes, as an essential part of medicine and pharmaceutical science, is required not only to gain a better understanding of liposomes pros and cons as drug carriers but also to confirm and evaluate them in clinical trials. The pharmacological properties of liposomal drugs and their interactions with the body can be examined in two various aspects:pharmacokinetic (the effect of the body on therapeutic compounds) and pharmacodynamics (how medications act and impact the body and cellular pathways) [73]. In general, the utilization of liposomes for drug delivery in cancer treatment or other disorders requires the elevation of these agents' effectiveness on the one hand, and reducing their toxicity toward normal tissues on the other hand. Subjects such as the proper administration route of NP-based drugs, their circulation in the bloodstream and half-life, their biological distribution in tissues, and their cellular metabolism, as well as their elimination, metabolization and clearance, have been studied in the field of pharmacokinetics [74]. The pharmacokinetics of liposomes primarily study the bioavailability of liposome-conjugated drugs in various body fluids and tissues. Indeed, the study of chemical decomposition and biological excretion, and liposome uptake and purification are also considered in pharmacokinetics. The results of studies on pharmacological advantages of using liposomal drugs (regardless of the type of liposomes applied in the DDS) instead of free drugs showed that:
Primarily, liposome can modify the drug release profile to a sustained release, and consequently, reduce the requirement for constant injection. Secondly, it can extend the presence of the drug in the bloodstream and body fluids, and as a consequence, increase its half-life. Thirdly, it has the potential to lead to better bio-distribution in cancerous tissues while reducing drug influences on healthy tissues due to limited particle size to cross the Endothelium of healthy capillaries. Ultimately, it reduces drug metabolism and inactivation in plasma before reaching the target tissue, in addition to its positive effect on the clearance of drug metabolites [75, 76].
However, some changes are required in the pharmacokinetics of liposomes to increase their solubility, specificity, and sensitivity. These modifications enable them to overcome chemotherapy-resistant cells, enhance the efficacy and half-life. Moreover, their toxicity or unintended metabolic compounds product as a result of their metabolization should be decreased by these modifications [77].
After consuming liposomal drugs administration, they enter the body and circulate in the bloodstream with a specific half-life. Their size and formative composition determine the half-life of liposomal medications. Moreover, rapid clearance of liposomal drugs from the body can reduce their duration of action and therapeutic index. As aforesaid, appending hydrophilic polymers such as PEG to liposomes is able to decrease their clearance rate and solve this challenge [78]. Also, it is possible to adjust the fluidity and drug-release rate of the liposome membrane by adding cholesterol molecules.
The application of liposomes for drug delivery may lead to some changes in drug pharmacokinetics [79]. The ability of liposomes to change the pharmacokinetic properties of the various drugs and medications is one of their significant benefits in drug delivery systems [80]. Concerning the process of liposome clearance and elimination, it is obvious that liposomal structures are affected by plasma proteins after being administered. For instance, after injection of liposomal nanoparticles opsonins are adsorbed on the surface of the liposomes. Opsonins are plasma protiens which mostly include immunoglobulins and fibronectin [42]. Opsonins presence on the surface of liposomes will result in their elimination by MPS, as one of the significant elimination section of various drugs from blood and body fluids. They also clear liposomes through the attachment of some receptors such as complement C3b and Fc to opsonins-liposomes complex [81]. Various tissues and cells such as liver kupffer cells, macrophages present in the spleen, bone marrow, and lymph nodes are involved in the clearance of liposomal NPs [82].
According to the International Union of Pure and Applied Chemistry (IUPAC) definition, pharmacodynamics refers to the study of the pharmacological impact of compounds on living systems and the biochemical and physiological consequences of these effects [83]. The increased elusion to identify therapeutic agents when encapsulated in liposomes has been recognized as one of the pharmacodynamical benefits of liposomes utilization [84].
Furthermore, the physicochemical characteristics have significant influences on the pharmacology of liposomal drugs. The particle size, electrical charge of membrane, and the composition of membrane lipids are some of these physicochemical properties that can affect the pharmacokinetics and pharmacodynamics of the agents. Firstly, there is a direct relationship between the particle size of nanoparticles, including liposomes, and their clearance rate. By increasing the size of NPs, their elimination rate by the immune system and MPS cells will also enhance [85]. Secondly, it is worth mentioning that the net charge of liposome membranes is a consequence of the electrical charge of phospholipids and their other constituting particles that made them up. As a result, a rise in the membrane charge is associated with enhanced clearance rates of these agents [86]. The composition of membrane lipids and other structural features (such as hydrophilic core radius) also remarkably affect the pharmacokinetics of liposomal drugs [87].
More importantly, it has been hypothesized that different types of liposomes exhibit distinct drug kinetics/dynamics depending on their various structures. The drug release rate also rests on the number of phospholipid bilayers and the content of loaded drug compounds. It is also contingent upon the hydrodynamic diameter, total volume, and other pharmacokinetic properties as well [88].
Administration Route of Liposomal Drugs
Like many different drugs, NP-based liposomal medicines can be administered from a wide variety of routes. In other words, oral consumption [89] and distinct injection methods such as intravenous (I.V.) administration and various local injections are among the common administration routes of liposomal drugs [90]. The usage of nanoparticles, including liposomes, for drug delivery via oral administration has been highlighted as an effective strategy since the nanoparticles increase the bioavailability of medicines, improve their interaction with cells, and prevent any modifications in the molecular structure of the drug due to enzymes and gastric juices in the gastrointestinal tract. Moreover, they have the ability not only to enhance the release of remedial molecules into the mucosal and epidermal layer but also to protect drugs from unwanted changes during the first pass effect [89]. Intravenous injection is used as the primary administration route for many liposomal drugs approved by the FDA or other authorities [42]. On the other hand, subcutaneous (S.C.), intradermal (I.D.), intraperitoneal (I.P.), and intramuscular (I.M.), classified under the title of the local injection, are also utilized for administration of liposomal drugs [90,91,92].
Liposomal Drugs Fate In Vivo and Their Targeting Mechanism of Action
Following administration of the liposomal drugs, they reach the pathological lesions at the target site through the bloodstream and accumulate there. The mechanism of action of liposomal drugs on tumors starts with their accumulation at the target site, uptake of them by tumor cells, and the release of free drugs [93]. Subsequent to entering the body, liposomal drugs reach the tumors through various targeting mechanisms of action and then interact with cells in different ways [94]. In general, tumor-targeting mechanisms are divided into two categories:passive and active targeting. Passive targeting refers to the mechanisms in which liposomes are spontaneously accumulated at the tumor site and interact with target cells without the presence of a specific ligand [95]. The effect of enhanced permeability and retention (EPR) has been suggested as the most critical passive targeting mechanism. To be precise, the spontaneous accumulation of therapeutic NPs and liposomal drugs at the tumor site is called the EPR effect [96]. This phenomenon can be assigned to the leaky nature of tumor tissue vessels, unlike normal tissue capillaries, which makes them permeable to molecules and NPs. Consequently, This ultimately leads to the accumulation of drug compounds in these tissues and the effect of EPR [97]. The ultimate fate of the drug in the intracellular fluid and cytoplasm of tumor cells depends on several factors such as release mechanism, nanocarrier constituents, and molecule structure [98]. In healthy tissue, the number and the shape of capillaries are proportionate and normal, respectively. However, in cancerous organs, unlike healthy tissue, the number and the structure of capillaries are higher and deformed, respectively, because of the angiogenesis process. Moreover, the tumor capillaries structure is destroyed, and the endothelial phalanx cells are diminished. As a result, the volume of plasma fluid leaking into the intercellular space will be enhanced. In healthy tissue, however, capillary phalanx cells retain cellular tight adhesions, preventing NPs, small molecules, and liposomal drugs from seeping into the intercellular space [99]. The EPR effect in cancerous capillaries and their difference with normal and healthy tissue vessels are illustrated in Fig. 7.
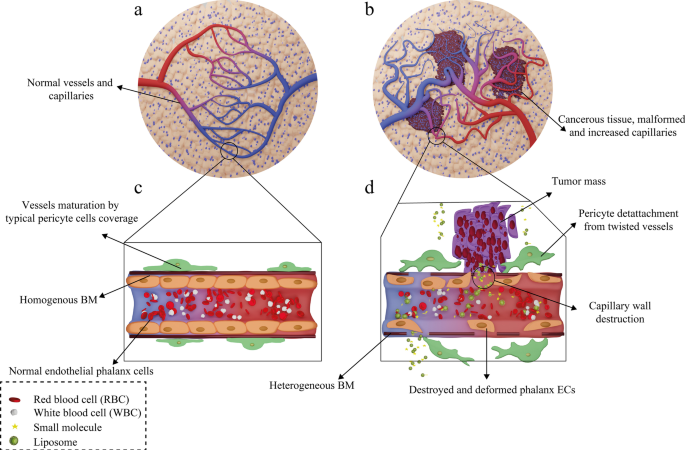
Mechanism of action of the drug-containing liposomes on tumor cells via EPR effect. een Healthy tissue and its normal capillaries; b cancerous tissue with increased-deformed vessels; c structure of normal and healthy vessel; d destructions and deformed capillary in tumor tissue
On the other hand, active targeting has attracted considerable attention as one of the targeting mechanisms of action owing to its appropriate effectiveness and high specificity. Active targeting includes various types and is also generally aimed to reduce the off-target impacts of liposomal NPs on healthy cells and non-target tissues [95]. In this method, molecules such as monoclonal antibodies, small molecules, signal peptides, vitamins, particular carbohydrates, glycolipids, or aptamers are generally utilized for surface modification of liposomes [100, 101]. Moreover, active targeting can be split into various subtypes according to diverse features. For instance, it can be classified into two general categories:
- 1.
Targeting tumor cell and cancer tissue receptors:This method relies on conjugating specific molecules to the membrane surface of liposomes, making them able to bind to special or overexpressed receptors on cancer cells [102]. In cancer cells, upregulation of different genes causes an increase in the expression of specific cell surface receptors in response to enhanced metabolic demands for rapid cell proliferation [103]. In active targeting, particular molecular modifications can be applied for targeting specifically the overexpressed surface receptors of cancer cells, such as folate receptor (FR), transferrin receptor (TfR), or Epidermal growth factor receptor (EGFR) [95]. In this regard, the role of folate receptors in cancer cells is to increase folic acid uptake [104], whereas transferrin receptors bind to transferrin (as a free molecule with 80 kDa weight in serum) and cause endocytosis of this monomeric glycoprotein to occur [105]. Moreover, EGFR receptors are a class of tyrosine kinases involved in cellular processes such as tissue differentiation and repair. The expression of this receptor in cancer cells is significantly increased due to its involvement in processes such as angiogenesis, cell proliferation, and metastasis [106].
- 2.
Utilizing tumor microenvironment as the target:In this method, changes in the surface of liposomes are exploited to enable them to target signal peptides or other receptors in the microenvironment of cancer cells. In other words, this active targeting mechanism can inhibit the growth of tumor cells and metastasis, prevent genotypic and phenotypic variations in neovascular endothelial cells, and control drug resistance [107]. Furthermore, some receptors in the tumor microenvironment, such as Vascular endothelial growth factor (VEGF), Vascular cell adhesion protein (VCAM), matrix metalloproteases, and integrin, are targeted in this mechanism [95].
Cellular Uptake of Therapeutic NPs and the Effect of Liposomal Drugs on Targeted Cells:Actions and Interactions
As it is mentioned earlier, liposomes are able to target tumor cells either passively or actively. After the liposome reaches the cancerous cells and the tumor environment through the targeting mechanism, it can release its therapeutic content and exert its effects by means of various mechanisms. Consequently, lipid composition, the surface charge of the membrane, type of cancer, type of target cells, as well as the presence of specific ligands on the liposome membrane, can influence the cell-liposome interaction [108].
Figure 8 illustrates different types of liposomes interactions with target cells. After being injected into the body, drug-containing liposomes travel to different tissues through blood vessels and eventually reach their target cells based on their surface ligands. These liposomes can bind to cellular receptors via these ligands, which is called specific absorption [42]. Albeit, receptor-free liposomes can also adhere to the target cell surface through molecular attractions, electrostatic forces, and molecular interactions called non-specific absorption. Following liposomes binding to the cell, the therapeutic agent is released into the cytoplasm, and its effects may be produced in different ways. The liposomal nanocarriers can be entirely fused to the plasma membrane of the cell and release the drug. Drug compounds are also able to be released from the liposome into the cell and to enter the cell through micropinocytosis or passive diffusion without the occurrence of fusion. Liposomes may directly interact with the cell or exchange lipid fragments with the cell membrane through protein-mediated processes. At the same time, the drug may act on the cell and exert the therapeutic effects of the liposomal drug. However, some liposomes are capable of entering through endocytosis (specific or nonspecific). In particular, liposomes penetrating the cell via this passage can have various destinies. It is possible for them to combine with lysosomes. In such cases, lysosomal enzymes affect the structure of the drug by reducing the pH of the phagolysosome sac. Ultimately, liposomes release the drug by fusing it to the cell membrane or endocytosis, and after that, medications exert their therapeutic effect [42, 62]. All possible ways for the liposome to penetrate the cell and exert its effect are depicted and compared in Fig. 8.
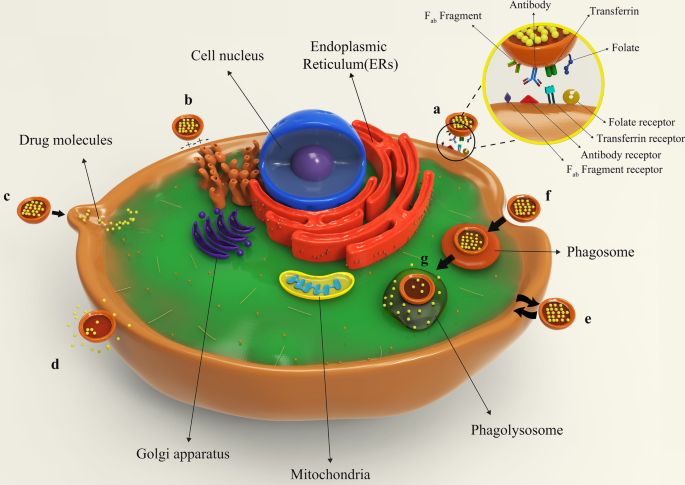
Binding of liposomes to the target cell. een Specific attachment via ligand-receptor interaction; b non-specific absorption of liposomes through intramolecular-electrostatic forces; c the attachment and fusion of liposome to the cell membrane and drug release; d liposome arrival to the target cell and drug release without fusion; e exchange lipid fragments between the cell membrane and liposome through protein-mediated processes; v endocytosis of liposome by target cell; g lysosomal digestion of liposome in the cell cytoplasm
On the other hand, NP-based medications can undergo endocytosis, pinocytosis, or phagocytosis by the target cells. Endocytosis is known as the process in which compounds outside the cell space approach the cell membrane and then enter the cell as a vesicle [109]. Pinocytosis, also recognized as fluid endocytosis, occurs when small molecules or suspensions are introduced into a cell through a vesicle by creating an invagination in the cell membrane. Moreover, pinocytosis vastly occurs in human cells to absorb fat droplets. In an immunological study, Yuriko Tanaka et al. reported that liposome-coupled antigens pinocytosis can be performed by antigen processing cells (APC). This report had proved that liposomes can undergo pinocytosis mechanisms [110]. In phagocytosis, particles larger than 0.5 μm are engulfed by immune cells, it may also occur for liposomes (especially for MLVs and liposomes larger than 500 nm). For example, Jitendra N. Verma et al. confirmed the occurrence of phagocytosis on liposomes by a study on the phagocytosis of liposomes with malarial antigens by macrophages [111].
Kaposi's Sarcoma, One Instance of Successful Liposomal Drugs Applications
Kaposi's sarcoma is a progressive multifocal anti-proliferative cancer primarily known as endometrial sarcoma. This cancer is more common in HIV patients whose immune system is weakened. Furthermore, it has been commonly seen in skin tissue and may also involve other tissues. Hence, this disorder is generally referred to as skin mucosal sarcoma [112]. To treat this disease, modified long-circulating liposomes can be helpful. In this regard, liposomes passively target tumor cells. Moreover, the effect of EPR and specific binding increases the concentration of the therapeutic drug in cancer tissues 5 to 11 times higher than normal skin [113]. For this purpose, Doxorubicin is used for the treatment of this disease. Correspondingly, entrapment of the doxorubicin into liposomes (which was PEGylated to prolong its half-life) prevents normal tissues from being exposed to the drug. It also reduces drug uptake by these healthy doxorubicin-sensitive tissues such as the heart [114].
Additionally, the liposomal form of doxorubicin, Doxil, is a type of anthracycline drug which is approved for clinical administration by US-FDA. It is used to treat AIDS-related Kaposi sarcoma and multiple myeloma [115]. Doxil has better therapeutic efficacy and less toxicity than free doxorubicin, which can be attributed to its ability to target tumors indirectly. It is also passive targeting due to leakage of tumor vessels and the EPR effect [116]. Moreover, the Doxil unilamellar liposomes are < 100 nm in size and have been used to treat various cancer types [42]. Analyses have also proved that free doxorubicin concentration is lower than that of Doxil at the target tissue site [117]. In this regard, Ogawara et al. investigated the effect of Doxil (formed by binding doxorubicin to PEG liposomes) on cancer cells in male mice and showed that PEG liposomal doxorubicin or Doxil1 had been effective on both doxorubicin-resistant and doxorubicin-sensitive C26 cell groups [118]. This can highlight the significance of the exploitation of liposomal NPs. Because they can be consumed to overcome the resistance of cancer cells to common chemotherapy agents at low costs without time-consuming research works to discover new clinical therapeutic compounds [119]. The application of nanoparticles, such as liposomes, to deliver doxorubicin to tumor tissues has been widely investigated. Entrapment of ATP-binding cassette transporter superfamily B member 1 (ABCB1) substrate doxorubicin into liposomes can increase drug uptake and enhance its intracellular distribution within cancer cells, especially ABCB1-expressing cancer cells [120]. The simple structure of Doxil is illustrated in Fig. 9.
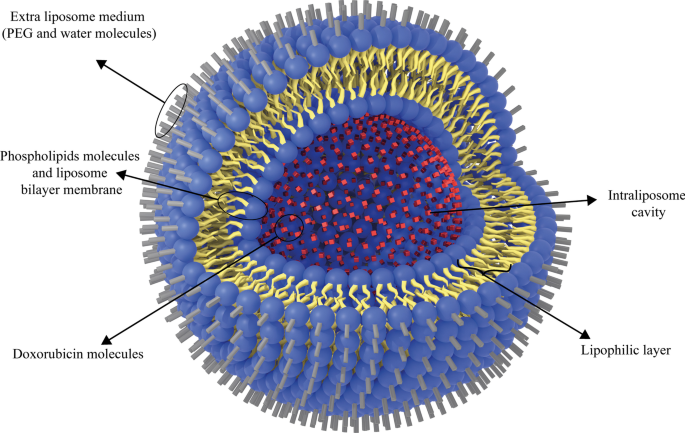
The schematic structure of Doxil drug. Doxorubicin drug molecules are entrapped in the hydrophilic cavity of unilamellar PEGylated liposomes
Furthermore, liposomal nanomaterials can be exploited for the treatment of infectious diseases. Systemic fungal infection is one of the most challenging conditions that is usually treated with amphotericin B, which is highly toxic to kidney cells. For this purpose, the usage of liposome-entrapped amphotericin B can reduce the toxicity of this drug compared to its free form [48]. Unilamellar liposomes have been used to entrap this agent. It has proven that liposomal amphotericin B is more effective than the free drug form [121]. Based on the formulation, these liposomes also alter the bio-distribution of amphotericin B, such as anticancer drugs, which in turn not only arrange the mechanism of action but also increase the effective dosage concentration at the target tissue [122]. AmbiSome, liposomal form amphotericin B, is approved for public administration too. Other approved liposomal drugs, from anti-fungal medications to cancer therapeutic agents, are summarized in Table 1.
Although the application of liposomal NPs to treat cancer has been touted as a viable solution for drug delivery and affecting tumor cells, drug delivery to cancerous tissues in the central nervous system (CNS) has remained a significant challenge. In addition, drug delivery to central nervous system cells faces many turbulences owing to a blood–brain barrier (BBB). However, this problem can be partially solved by developing new methods and using lipid-based compounds [136].
Liposomal Nanoparticles in the Investigational Phase for Therapeutic Purposes
Liposomal siRNA
RNA is a type of genetic molecule with a variety of functions, including translation and transcription processes. The discovery of small-interfering RNA (siRNA) is a significant advance in biology in the last decade [137]. Synthetic siRNAs can be utilized to target oncogenes and their mRNAs. Furthermore, siRNAs can be applied for targeting genes contributing to the carcinogenesis, proliferation, and metastasis of tumor cells or their resistance to standard chemotherapies and radiation [138]. Therefore, it has been considered a modern method for cancer therapy. On the other hand, the nanoparticles used to deliver siRNA must possess properties such as biodegradability, great bio-distribution, low toxicity, etc. All of these features can be offered by liposomes making this popular drug delivery system a promising candidate for this purpose [28]. siRNAs bound to neutral lipid-based NPs are well isolated from these liposomes. They also influence ephrin type-A receptor 2 (EphA2), focal adhesion kinase (FAK), neuropilin-2, Interleukin 8 (IL-8), and TROJAN Mobile Remote Receiving System/erythroblast transformation-specific (TMRRS/ERG), Elongation factor 2 kinase (EF2K) or Bcl-2 pathways. Following the occurrence of this mechanism, a suitable antitumor effect has been observed against ovarian, colon, and breast cancer cells, etc. [139, 140]. Numerous studies have been conducted on siRNA delivery by liposomes, and in most of them, the cationic lipid Dioleoyl-3-trimethylammonium propane (DOTAP) has been widely expended in the structure of liposomes. Due to DOTAP high positive charge, this cationic lipid can be toxic to cells. It can stimulate cellular hemolysis and reduce ultimate biocompatibility as well. This has challenged the application of this lipid in the composition of liposomes applied for siRNA delivery [141].
Liposomal Curcumin Nanoparticles
Curcumin-conjugated liposomes are another instance of liposomal nanoparticle usage. Curcumin is a natural polyphenolic and hydrophilic compound that is abundant in the Curcuma longa plant and can be mainly prepared from turmeric extraction. Nowadays, the anticancer effect of curcumin has been well indicated against many tumor cells, such as breast cancer, liver carcinoma, and prostate cancer, etc. [142]. The primary mechanism of action of curcumin against cancer cells is to interfere with the translation of proteins such as Bcl-xl and regulate apoptosis by influencing their process, controlling the release of reactive oxygen species (ROS) and cytochrome, regulating molecular factors such as cyclin affecting the cell cycle. On the other hand, curcumin can damage the nuclear and mitochondrial DNA structure of liver cancer cells, thereby disrupting their function [143]. In comparison with free curcumin, the application of liposomal curcumin improves pharmacokinetics and pharmacodynamics while reducing the dosage required to target tumors. Matheus Andrade Chave et al. explored curcumin-containing liposomes by inserting curcumin molecules into the MLV liposome [144]. The synthesis of liposomal curcumin and curcumin structure are described in Fig. 10.
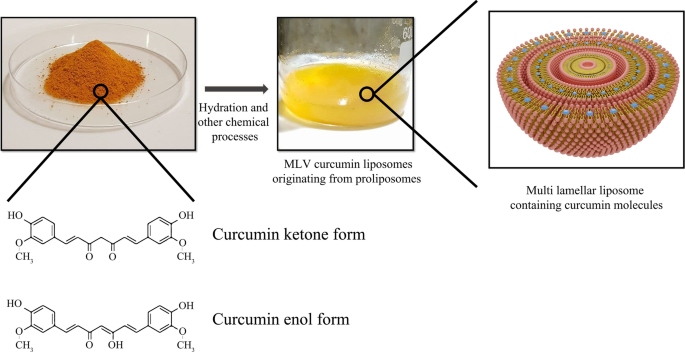
An overview of curcumin powder and liposomal curcumin synthesis. Chemical reactions performed for liposomal curcumin production and curcumin molecule structure in various forms are simply demonstrated
In addition, liposomes prepared for therapeutic research applications can be synthesized by employing various methods. For example, Qiao Wang et al. exploited the ultrasonication and lipid film-hydration method to synthesize daidzein long-circulating liposomes (DLCL) [145]. Xiaoyuan Ding et al. also used the film hydration method for the synthesis of aptamer and Au-NPs (Apt-Au)-modified Morin pH-sensitive liposome. Their outcomes showed high biocompatibility and insignificant toxicity of these liposomal structures and highlighted these liposomes as a viable option for selective targeting of tumors [146].
Other Liposomal NPs in the Investigational Phase
Several liposomal drugs have been synthesized and utilized in various medications at the investigational phase. For instance, CPX-1 was produced by entrapping the antitumor agents, Irinotecan and floxuridine (1:1 molar ratio) in liposomes, and was designed to treat advanced colorectal cancer. This therapeutic nanoparticle is in phase II research status [128]. Lipovaxin-MM is another momentous liposomal nanoparticle in phase I research prepared by placing melanoma antigens in liposomes and mainly administrated for immunotherapy of malignant melanoma. This agent is also under investigation [128].
Conclusie
As spherical structures in liquids, liposomes can be applied as a promising option for cancer therapy and drug delivery, as well as imaging, and disease management. By reviewing liposomes pros and cons, scientists will be able to improve them in future research works.
Some opportunities and challenges in liposomes utilization are described in the following. One of the convenient features of liposomes is their morphological similarity to cells (presence of phospholipids), as well as increasing the effectiveness of the drugs. As a negative point, liposomal phospholipids may sometimes undergo hydrolysis or oxidation reactions which may be problematic. Other pros of liposomes include increased stability of the encapsulated drug in it, reduced contact of sensitive tissues with therapeutic molecules, decreased drug toxicity, improved pharmacokinetic and pharmacodynamics properties, the ability to regulate the rate of drug release, and the potential of their structure to accept the desired chemical modification. In contrast to these opportunities, there are some challenges such as leakage or unintended entrapment of drugs, low liposome bioactivity, decreased-solubility, rapid clearance of conventional liposomes from the blood by the reticuloendothelial system (RES), and problems caused by continuous intravenous administration or local injection.
Besides examining the advantages and disadvantages of liposomes, we should take their proper targeting mechanism of action into account. Passive targeting is considered a beneficial mechanism due to the abundant clinical evidence and experience. It also increases the circulation time of liposomal drugs. The problem of this mechanism lies in its non-specific drug delivery and its physiological barriers. In contrast, beneficial features of active targeting include increased specificity in drug delivery, the possibility of overcoming chemotherapy-resistant tumor cells, and reduced off-target effects. However, the difficulty in identifying accurate binding sites on cancer cells and the lack of adequate evidence of its former utilization have led to some ups and downs in its application.
Liposomes are reasonable candidates for elevating the effectiveness of current anticancer agents and preventing the incidence of drug resistance. Future research in this area should be focused on further investigation into the properties of liposomal structures. To probe about drug entrapment in therapeutic nanoparticles, including liposomes, much more detailed examinations will be required.
Afkortingen
- WHO:
-
World Health Organization
- VNPs:
-
Viral nanoparticles
- NP's:
-
Nanodeeltjes
- US FDA:
-
United States Food and Drug Administration
- DDSs:
-
Drug delivery systems
- PC:
-
Fosfatidylcholine
- SM:
-
Sphingomyelin
- PS:
-
Phosphatidylserine
- PE:
-
Phosphatidylethanolamine
- ULVs:
-
Unilamellar vesicles
- OLVs:
-
Oligo lamellar vesicles
- MLVs:
-
Multilamellar vesicles
- SUVs:
-
Small unilamellar vesicles
- RBF:
-
Round-bottom flask
- LNs:
-
Liposomal nanoparticles
- MPS:
-
Mononuclear phagocytosis system
- PEG:
-
Polyethyleenglycol
- PEOZ:
-
Poly [2-ethyl 2-oxazoline]
- PETOXylated:
-
Poly [2-ethyl-2-oxazolylated
- PMOZ:
-
Poly [2-methyloxazoline]
- HDL:
-
High-density lipoprotein
- LDL:
-
Low-density lipoprotein
- SCLC:
-
Small cell lung cancer
- GRPR:
-
Gastrin-releasing peptide receptor
- Anti-EpCAM:
-
Anti-epithelial cell adhesion molecule
- IUPAC:
-
International Union of Pure and Applied Chemistry
- I.V.:
-
Intravenous
- S.C.:
-
Subcutaneous
- I.D.:
-
Intradermal
- I.P.:
-
Intraperitoneal
- I.M.:
-
Intramuscular
- EPR:
-
Verbeterde doorlaatbaarheid en retentie
- fR:
-
Folate receptor
- TfR:
-
Transferrin receptor
- EGFR:
-
Epidermale groeifactorreceptor
- VEGF:
-
Vascular endothelial growth factor
- VCAM:
-
Vascular cell adhesion protein
- APC:
-
Antigen-presenting cells
- ABCB1:
-
ATP-binding cassette transporter superfamily B member 1
- HSPC:
-
Hydro soy phosphatidylcholine
- DSPG:
-
1,2-Distearoyl-sn-glycero-3-PG
- DOPC:
-
Dioleoylphosphatidylcholine
- DPPG:
-
1,2-Dipalmitoyl-sn-glycero-3-phosphoglycerol
- DSPC:
-
1,2-Distearoyl-sn-glycero-3-phosphocholine
- AML:
-
Acute myeloid leukemia
- ALL:
-
Acute lymphocytic leukemia
- DSPE:
-
1,2-Distearoyl-sn-glycero-3-phosphoethanolamine
- DOPE:
-
Dioleoylphosphatidylethanolamine;
- EPG:
-
Esterified propoxylated glycerols;
- DMPC:
-
1,2-Dimyristoyl-sn-glycero-3-phosphocholine
- DOPS:
-
1,2-Dioleoyl-sn-glycero-3-phospho-L-serine
- POPC:
-
1-Palmitoyl-2-oleoyl-sn-glycero-3-phosphocholine
- DMPG:
-
1,2-Dimyristoyl-sn-glycero-3-phosphoglycerol
- MPEG:
-
Methoxypolyethylene glycols.
- CNS:
-
Central nervous system
- BBB:
-
Blood–brain barrier
- siRNA:
-
Small-interfering RNA
- EphA2:
-
Ephrin type-A receptor 2
- FAK:
-
Focal adhesion kinase
- IL-8:
-
Interleukin-8
- TMRRS/ERG:
-
TMRRS/ERG TROJAN Mobile Remote Receiving System/erythroblast transformation-specific
- EF2K:
-
Elongation factor 2 kinase
- DOTAP:
-
Dioleoyl-3-trimethylammonium propane
- ROS:
-
Reactieve zuurstofsoorten
- DLDC:
-
Daidzein lang circulerende liposomen
- RES:
-
Reticuloendothelial system
Nanomaterialen
- Correcte pompselectie essentieel voor zaadbehandelingstoepassingen
- Nanovezels en filamenten voor verbeterde medicijnafgifte
- Nanodeeltjes voor kankertherapie:huidige vooruitgang en uitdagingen
- Omgevingsgevoelige metaal-organische raamwerken als medicijnafgiftesysteem voor tumortherapie
- Op cellen gebaseerde medicijnafgifte voor kankertoepassingen
- Zebravis:een veelbelovend real-time modelsysteem voor door nanotechnologie gemedieerde neurospecifieke medicijnafgifte
- 131I-getraceerde PLGA-lipide nanodeeltjes als dragers van medicijnafgifte voor de gerichte chemotherapiebehandeling van melanoom
- UV-behandeling van flexibele koperen nanodraadgaasfilms voor toepassingen met transparante geleiders
- Nucleoside-lipide-gebaseerde nanocarriers voor toediening van sorafenib
- 5-aminolevulinezuur-squaleen nanoassemblages voor tumorfotodetectie en therapie:in vitro studies
- 3D-geprinte microrobots houden belofte voor medicijnafgifte