In water oplosbaar fullerenol met hydroxylgroepafhankelijkheid voor efficiënte twee-foton opgewekte fotodynamische inactivatie van infectieuze microben
Abstract
We hebben met succes in water oplosbaar fullerenol [C60 . gemaakt (OH)46 ] die een hoge kwantumopbrengst van singletzuurstof vertoonde en efficiënt gegenereerde reactieve zuurstofsoorten. Bovendien is de in water oplosbare C60 (OH)46 met een hogere samenstelling van blootgestelde hydroxylgroepen had superieure stabiliteit en eigenschappen van twee fotonen vergeleken met die met een lagere samenstelling van dergelijke groepen. Daarom kan het bereide fullerenol een effectieve fotosensibilisator met twee fotonen zijn. De in water oplosbare C60 (OH)46 had gunstige twee-foton eigenschappen. Tijdens fotodynamische therapie met twee fotonen wordt de in water oplosbare C60 (OH)46 substantiële antimicrobiële activiteit had tegen Escherichia coli op een ultralaag energieniveau van 211,2 nJ pixel −1 met 800 scans en een foto-exciteerde golflengte van 760 nm.
Inleiding
De afgelopen decennia zijn er verschillende fotosensitizer (PS) moleculen gesynthetiseerd [1]. Klinische toepassingen van bestaande PS's brengen echter verschillende problemen met zich mee. De meeste PS-moleculen zijn hydrofoob en kunnen gemakkelijk aggregeren in waterige media, waardoor hun kwantumopbrengst (QY) [2] wordt verminderd. Bovendien kunnen geaggregeerde PS's niet zomaar intraveneus worden geïnjecteerd. Selectieve accumulatie van PS-moleculen in overleden weefsels is ook vereist om schade aan gezonde cellen te voorkomen. Vanwege deze problemen blijft het ontwikkelen van een effectieve PS-drager een grote uitdaging voor fotodynamische therapie (PDT). Dienovereenkomstig neemt de belangstelling voor het gebruik van nanodeeltjes als PS-dragers toe.
Vooruitgang in nanobiotechnologie heeft de interesse gestimuleerd in de biomedische toepassingen van een nieuwe klasse van nanostructuren [3,4,5,6,7,8,9,10,11] die uitsluitend uit koolstofatomen zijn samengesteld, namelijk fullereen C60 , die bolvormige moleculen zijn (0,72 nm in diameter) die niet-toxisch zijn en unieke fysisch-chemische eigenschappen hebben. De kleine omvang van lipofiele C60 moleculen is verantwoordelijk voor hun sterische compatibiliteit met biologische moleculen en bevordert hun integratie in hydrofobe gebieden van membranen [12, 13]. Vanwege de uitgebreide π -geconjugeerd systeem van zijn moleculaire orbitalen, fullereen C60 absorbeert ultraviolet-zichtbaar (UV-vis) licht en kan reactieve zuurstofsoorten (ROS) genereren met een bijna 100% singlet zuurstof QY (Φ Δ ). Verder zijn de fysisch-chemische eigenschappen van fullereen C60 het in staat stellen om ROS te genereren en als PS voor PDT te dienen. Fullerenen kunnen ook pro-oxidante effecten induceren, en dit kan worden bepaald door het gebruikte fullereen, het onderzochte celtype en de experimentele opzet [14,15,16,17]. C60 heeft een extreem lage oplosbaarheid in polaire oplossingen, wat de toepassingen in de geneeskunde aanzienlijk beperkt. Echter, vanwege de aanwezigheid van dubbele bindingen, C60 kan gemakkelijk worden gewijzigd met behulp van chemische groepen om de oplosbaarheid in water te vergroten. Daarom is in water oplosbaar C60 derivaten hebben de mogelijkheden voor medische toepassingen vergroot, waaronder neuroprotectie, medicijn- en genafgifte, fotosensibilisatie en biosensing.
Multiphoton lasermicroscopie (ook bekend als twee-foton lasermicroscopie) omvat het gebruik van gelokaliseerde "niet-lineaire" excitatie om fluorescentie op te wekken in slechts een dun raster-gescand vlak. Twee-foton lasermicroscopie is gebruikt in verschillende beeldvormende onderzoeken [18]. Het wordt meestal gekoppeld aan nabij-infrarood (NIR) laserexcitatie om te profiteren van de inherente maximale weefseltransmissie voor biobeeldvorming; dit komt omdat NIR de voordelen heeft van lichte verstrooiing, lage energieabsorptie, optimale bestralingspenetratie en verminderde fotobleking van specimens. De koppeling van twee-fotonenlasermicroscopie met NIR-laserexcitatie is de voorkeurstechniek geworden voor fluorescentiemicroscopie in dik weefsel en diepere biologische monsters [19, 20] en is uitgebreid toegepast in andere foto-excitatietherapieën [21, 22]. Bovendien wordt, vanwege de ultralage energie en korte foto-excitatie, twee-foton lasermicroscopie beschouwd als een alternatieve benadering voor het uitvoeren van PDT [23]. Hoewel sommige PS's giftig zijn [24, 25], zijn die met een hoge Φ Δ krijgen prioriteit bij het uitvoeren van PDT. Een hoge Φ Δ waarde is met name wenselijk wanneer twee-fotontechnieken worden gebruikt om moleculaire activiteiten in foto-eigenschappen te evalueren en om niet-lineaire microscopische studies efficiënt uit te voeren; een dergelijke waarde is wenselijk omdat de verhouding van de geabsorbeerde energie tot de input-energieflux naar een monster hoog is, waardoor mogelijke fotoschade aan het monster wordt geminimaliseerd [26]. De literatuur bevat echter geen studies die het gebruik van materialen met twee-fotoneigenschappen voor PDT hebben overwogen. Om deze onderzoeksleemte op te vullen, werd in de huidige studie in water oplosbaar gehydroxyleerd fullerenol toegepast met een sterk elektronendonatievermogen en een grote π -geconjugeerd systeem om de efficiëntie van de ladingsoverdracht te verhogen, waardoor de eigenschappen van twee fotonen worden verbeterd. Specifiek, in water oplosbaar gehydroxyleerd C60 (OH)46 werd afgeleid en toegepast als een twee-foton PS voor effectieve verwijdering van microben met behulp van ultralage energie femtoseconde laserbestraling en slechts 800 scans onder twee-foton-excitatie (TPE; excitatiegolflengte, 760 nm). Voor de femtoseconde laserbestraling met ultralage energie was de energie 211,2 nJ pixel −1 en het vermogen was 2,112 mW (betreffende de berekening van het laservermogen na het doel, zie de sectie "Materialen en methoden" en Fig. 1a, waar de x –j brandpunt en z -asresolutie van het lasersysteem is respectievelijk ongeveer 0,37538 en 0,90159 m); bovendien was de totale effectieve belichtingstijd voor het scanproces ongeveer 3,2621 s, de scansnelheid 4,0776 ms scan −1 , en het scangebied was 200 × 200 μm 2 (zie de sectie “Materialen en Methoden” voor details over de berekening). Het in water oplosbare gehydroxyleerde C60 (OH)46 bereikte een bijna 100% eliminatie van Escherichia coli (E. coli , een Gram-negatieve bacteriestam). Verder is de in water oplosbare C60 (OH)46 met een hogere samenstelling van hydroxylgroepen vertoonde superieure foto-eigenschappen van twee fotonen vergeleken met die met een lagere samenstelling van hydroxylgroepen onder TPE; daarom is de afgeleide in water oplosbare C60 (OH)46 kan worden beschouwd als een aanzienlijk potentieel voor gebruik bij gelijktijdige PDT om kwaadaardige microben te elimineren.
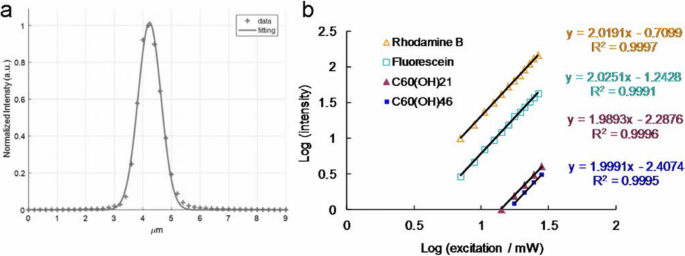
een Volgens de z -axis scan van een gouden dunne film die wordt gebruikt om het signaal van de tweede harmonische generatie op verschillende posities te meten, de z -asresolutie van het lasersysteem (volledige breedte op halve maximum) is ongeveer 0,90159 μm (passend met behulp van de Gauss-functie). b TPL-intensiteitsafhankelijkheid van het excitatievermogen (logaritme) van de materialen en fluoroforen; TPE-belichting van 704.0 tot 2816.0 nJ pixel −1 voor Rhodamine B en fluoresceïne, van 1408.0 tot 2816.0 nJ pixel −1 voor in water oplosbare C60 (OH)21 fullerenol, en van 1760,0 tot 2816.0 nJ pixel −1 voor in water oplosbare C60 (OH)46 fullerenol. Excitatiegolflengte, 760 nm. Afgeleverde dosis:OD600 0,05 van E. coli en 3 μg mL −1 materialen. Gegevens worden weergegeven als gemiddelden ± SD (n =6)
Materialen en methoden
Bereiding en karakterisering van in water oplosbare fullerenolen, C60 (OH)21 en C60 (OH)46 [27]
Ruw fullereen werd commercieel verkregen (Sigma-Aldrich, St. Louis, MO, VS) en de C60 (OH)12 voorloper werd geproduceerd, zoals eerder beschreven. Eerst werd 30% waterstofperoxide-oplossing (100 mL; Sigma-Aldrich, St. Louis, MO, VS) toegevoegd aan het uitgangsmateriaal, 0,5-1,0 g C60 (OH)12 en het mengsel werd onder lucht bij 60°C krachtig geroerd. Na afkoeling werd een mengsel van oplosmiddelen bestaande uit 2-propanol, diethylether en hexaan (100-200 mL voor elk; Sigma-Aldrich, St. Louis, MO, VS) aan de oplossing toegevoegd, die vervolgens werd gecentrifugeerd en gedecanteerd. De overblijvende vaste stof werd tweemaal gewassen met 200 ml diethylether door middel van centrifugatie- en decantatieprocedures. Ten slotte zijn de eindproducten van in water oplosbaar C60 (OH)21 en C60 (OH)46 werden verkregen door het residu respectievelijk een nacht onder vacuüm bij kamertemperatuur te drogen. Het gewicht van het eindproduct werd gekalibreerd door middel van thermische gravimetrische analyse. De morfologie van het eindproduct werd waargenomen met behulp van een transmissie-elektronenmicroscopie met hoge resolutie (HR-TEM, JEOL 3010, Akishima, Tokyo, Japan) met een resolutie van ongeveer 1,11 ± 0,03 nm en 1,13 ± 0,04 nm voor C60 (OH)21 en C60 (OH)46 , respectievelijk. De dynamische lichtverstrooiing (DLS, Malvern Nano-ZS90, Worcestershire, West Midlands, VK) werd ook gebruikt om de grootte van materialen te bepalen. De blootgestelde functionele groepen van de bereide materialen werden eerst onderzocht door middel van Fourier-transform infrarood (FTIR) spectroscopie (RX1, PerkinElmer, Waltham, MA, VS). UV-vis-spectroscopie van de materialen werd uitgevoerd met behulp van een spectrometer (U-4100, Hitachi, Chiyoda-ku, Tokyo, Japan). De oppervlaktechemie van het fullerenol werd onderzocht door middel van röntgenfoto-elektronspectroscopie (XPS, PHI 5000-spectrometer (VersaProbe, Chanhassen, MN, VS)). Het molecuulgewicht van fullerenol werd bepaald met behulp van een velddesorptie (FD) massaspectrometer (AccuTOF, GCx-plus, JEOL, Akishima, Tokyo, Japan), en het aantal hydroxylgroepen werd bevestigd op 21 en 46 op basis van de resultaten, respectievelijk.
Bacteriële culturen [28]
E. coli , verkregen uit ons eigen laboratorium werden gekweekt in voedingsagar van LB (per liter:trypton 10 g, gistextract 5 g, natriumchloride 8 g, agar 15 g en pH afgestemd op 7,5) (Sigma-Aldrich, St. Louis, MO, VS) en geïncubeerd bij 37 °C.
Biocompatibiliteitstest met telmethode voor kolonievormende eenheden (CFU) [28]
E. coli (OD600 ~ 0.05) is toegevoegd met materiaal (0–9 μg mL −1 ), en gedurende 3 uur bij 37 ° C geïncubeerd (aanvullend bestand 1:Fig. S1). Na incubatie werd het mengsel gecentrifugeerd en werden de bacteriepellets verdund (OD600 ~ 0.05). Een verdunningsfactor van 10 −5 tot 10 −8 werd vervolgens uitgevoerd in de geïncubeerde bacteriën en uitgeplaat op de agarplaten. De platen blijven een nacht in een incubator (bij 37°C). Het aantal overlevende bacteriën werd bepaald en uitgedrukt als een percentage (%) dat overeenkwam met de eenheid KVE ml −1 na incubatie. Gegevens zijn gemiddelden ± SD (n =6).
ψ Δ Meting [29, 30]
Volgens de vorige studie, ψ Δ kan worden verkregen. ψ Δ metingen werden uitgevoerd in D2 O op 355 nm, met behulp van meso -tetra(4-sulfonatofenyl)porfine dihydrochloride (TSPP; Sigma-Aldrich, St. Louis, MO, VS) als referentie (ψ Δ =0,64).
Fluorescentie QY-meting [31, 32]
De relatieve fotoluminescentie (PL) QY van contrastmiddel is meestal de verhouding van de uitgezonden fotonen tot de geabsorbeerde fotonen en wordt als volgt gegeven:
$$ \mathrm{QY}={\mathrm{QY}}_{\mathrm{ref}}\ \left({\eta}^2/{\eta_{\mathrm{ref}}}^2\right) \left(I/A\right)\left({A}_{\mathrm{ref}}/{I}_{\mathrm{ref}}\right) $$ (1)waar QYref =0,28 is de QY van Cy5.5 opgelost in dimethylsulfoxide (DMSO; Sigma-Aldrich, St. Louis, MO, VS) als referentie, η is de brekingsindex van ddH2 O =1,33 (η ref van DMSO =1,48), I is de geïntegreerde fluorescentie-intensiteit en A is de absorptie bij de excitatiegolflengte. One-photon excitation (OPE) of TPE levert dezelfde QY op.
Femtoseconde optische lasersysteem voor de metingen van twee-fotonabsorptie (TPA) en twee-fotonluminescentie (TPL) [23, 28, 33,34,35 ,36,37,38]
Het zelfgemaakte femtoseconde titanium-saffier (ti-sa) optische lasersysteem (een herhalingssnelheid van 80 MHz; Tsunami, Spectra-Physics, Santa Clara, CA, VS) werd gebruikt volgens de eerdere onderzoeken.
TPA-meting
Met een galvanometer-scannersnelheid van 2 m ms −1 , het excitatiespectrum werd gemeten als 720-820 nm met een excitatievermogen van 2,8 mW [dit is het vermogen vóór het objectief; het vermogen na objectief (of op monster) is 0,9856 mW of 98,56 nJ pixel −1 ]. Daarom werden de relatieve TPA-spectra als functie van de excitatiegolflengte voor de fullerenolen gemeten.
Meting van TPL-spectra
Het materiaal werd blootgesteld aan TPE van de femtoseconde laser bij een excitatiegolflengte van 760 nm, een scangebied van 200 × 200 μm 2 , een frequentie van 10 kHz, een belichtingstijd van 1,638 s/(scan, pixel) =100 μs, 128 × 128 pixels scan −1 , en een pixeloppervlak van 1562,5 × 1562,5 nm 2 . Het brandpuntgebied werd berekend als πd 2 /4, waar d =0,61 λ/numeriek diafragma (NA ) is de volledige breedte op het halve maximum van de balktaille. Bijvoorbeeld bij de x –j as brandpunt met 760 nm excitatie en een × 40 olie-immersie objectief met een NA van 1.3, d =0,61 × 800 nm/1,3 =375,38 nm =0,37538 μm, en de z -as resolutie werd gemeten als 0,90159 μm. Voor 760 nm-excitatie wordt de belichtingstijd per scan voor een individueel nanomateriaal uitgedrukt als (brandpuntgebied/pixelgebied) × 100 =4,0776 ms, en de totale belichtingstijd t =4,0776 ms × aantal scans. Een × 40 olie-immersie objectief (NA 1.3) werd gebruikt om de signalen te verzamelen en het detectiebereik van de spectrumfotometer was 300-695 nm.
Bovendien kunnen de berekeningen van het laservermogen (mW of nJ pixel −1 ) die op het monster werden gebruikt, waren als volgt. Voor het × 40 olie-immersie objectief (NA 1.3), is de transmissiesnelheid bij 760 nm in golflengte ongeveer 88% in dit optische systeem, en het laservermogen ging van de output naar het objectief met slechts 40% van het originele outputvermogen als gevolg van het verlies van vermogen. Als resultaat is de berekende energie na het objectief (op monster) P uitvoer (mW)*40%*88% =0,352 × P uitvoer (mW). Bijvoorbeeld P uitvoer =2,8 mW, de berekende energie na het objectief (op het monster) is 3,0 mW * 40% * 88% =0,9856 mW. Met een scansnelheid van 10 kHz (elke puls blijft 0,1 ms pixel −1 ), de berekende energie op het monster (J pixel −1 ) was rond P uitvoer (mW)*40%*88%*0.1 ms =0.0352*P uitvoer (J pixel −1 ). Bijvoorbeeld P uitvoer =2,8 mW, de energie (J pixel −1 ) op monster =2,8 mW*40%*88%*0,1 ms =0,09856 μJ pixel −1 =98,56 nJ pixel −1 . De kracht na het objectief (op het monster) werd gebruikt en markeerde de doorvoer van dit manuscript.
Meting van TPE Absolute doorsnede [24, 36,37,38,39,40,41,42,43,44,45,46,47, 48]
De absolute dwarsdoorsnede van TPE werd gemeten met het luminescentiesignaal via femtoseconde laser optisch systeem volgens eerdere studies. De TPL van fluoresceïne en rhodamine B (Sigma-Aldrich, St. Louis, MO, VS) moest worden geverifieerd. De resultaten worden getoond in Fig. 1b en werden verkregen door de afhankelijkheid van de emissie-intensiteit te meten met een excitatievermogensbereik van 704 nJ pixel −1 (7,04 mW) tot 2816 nJ pixel −1 (28,16 mW). Kwadratische afhankelijkheid met de exponenten van 2,03 voor fluoresceïne en 2,02 voor rhodamine B werd gemeten voor het verhogen van het excitatievermogen om de luminescentie van TPE te bepalen. Volgens eerdere studies zijn de actiedwarsdoorsneden van TPE voor fluoresceïne en rhodamine B 36,4 en 68,0 GM (1 GM =10 −50 cm 4 s foton −1 ), respectievelijk voor 760 nm excitatie. We verwezen ook naar de gratis website http://www.drbio.cornell.edu/cross_sections.html, vriendelijk ter beschikking gesteld door Prof. Chris Xu (Cornell University, NY, VS). De TPE-actiedoorsneden voor fluoresceïne en rhodamine B werden berekend op respectievelijk 36,5 en 66,1 GM (tabel 1), wat een fout van minder dan 5% aangaf in vergelijking met die van het laboratorium van prof. Xu. In deze studie werd rhodamine B gekozen als de standaardreferentie voor het bepalen van de doorsnede, en de berekende absolute doorsneden van TPE voor de in water oplosbare C60 (OH)21 en C60 (OH)46 fullerenolen waren respectievelijk ongeveer 1230,51 GM en 1037,21 GM. De gemeten parameters voor het berekenen van de absolute TPE-doorsneden van monsters worden weergegeven in Tabel 3. Er werd geen batch-tot-batchvariatie waargenomen voor de materialen in twee-fotoneigenschappen en twee-foton fotodynamisch vermogen.
Femtoseconde laseroptisch systeem (voor fluorescentie lifetime imaging microscopie, FLIM) [39, 45]
Het zelfgemaakte femtoseconde ti-sa optische lasersysteem (herhalingssnelheid van 80 MHz; Tsunami, Spectra-Physics, Santa Clara, CA, VS) werd gebruikt volgens de eerdere onderzoeken. De levensduurgegevens en parameter worden gegenereerd met behulp van de fitting met drievoudige exponentiële vergelijking terwijl de emissie onder TPE (Ex, 760 nm) wordt bewaakt.
Berekening van stralings- en niet-stralingsvervalpercentages [46]
PL QY en levensduur zijn beide belangrijke parameters bij het onderzoeken van de emissiekenmerken van fluorescerende kleurstoffen in diverse omgevingen. De QY (Q ) kan als volgt worden uitgedrukt:
$$ Q=\frac{\varGamma }{\varGamma +k} $$ (2)waar Γ is de stralingsvervalsnelheid, en k is de niet-stralingsvervalsnelheid. De levensduur van fluorescentie wordt gewoonlijk gedefinieerd als de gemiddelde tijd die een elektron in de aangeslagen toestand nodig heeft om te vervallen tot de grondtoestand. De TPL-levensduur τ kan ook relatief zijn aan de vervalsnelheden en wordt als volgt beschreven:
$$ \tau =\frac{1}{\varGamma +k} $$ (3)Volgende vergelijkingen. (2) en (3), de stralings- en niet-stralingsvervalsnelheden kunnen worden berekend.
Bij de absorptie van een foton wordt een van de zwak gebonden elektronen van het fluorescerende molecuul - een fluorofoor - naar een hoger energieniveau gepromoveerd. De fluorofoor is dan in een aangeslagen toestand, A* . Deze toestand is metastabiel; daarom zal de fluorofoor terugkeren naar zijn stabiele grondtoestand, A . Het kan dit ofwel met straling doen door een fluorescentiefoton uit te zenden hν
$$ A\ast ->A+ h\nu $$of niet-radiatief door de energie van de aangeslagen toestand als warmte te dissiperen:
$$ A\ast ->A+\mathrm{heat} $$De ontvolking van de aangeslagen toestand hangt af van de beschikbare de-excitatieroutes. Fluorescentie is stralingsdeactivering van het laagste trillingsenergieniveau van de eerste elektronisch aangeslagen singlettoestand, S 1 , terug naar de elektronische grondtoestand, S 0 . De singlet-toestanden zijn de energieniveaus die kunnen worden bevolkt door het zwak gebonden elektron zonder een spin-flip. De absorptie- en emissieprocessen worden geïllustreerd door een energieniveaudiagram dat genoemd is naar Aleksander Jablonski.
De fluorescentielevensduur, τ , is de gemiddelde tijd dat een fluorofoor in de elektronisch aangeslagen toestand blijft S 1 na opwinding. τ wordt gedefinieerd als het omgekeerde van de som van de snelheidsparameters voor alle ontvolkingsprocessen in aangeslagen toestand:Vgl. (3), waarbij de niet-stralingssnelheidsconstante k is de som van de snelheidsconstante voor interne conversie k ic en de snelheidsconstante voor intersysteemovergang naar de triplettoestand k isc zodat k =k ic + k isc . Fluorescentie-emissie vindt altijd plaats vanaf het laagste trillingsniveau van S 1 , een regel die bekend staat als de Kasha-regel, wat aangeeft dat de fluorofoor geen herinnering heeft aan zijn excitatiepad; OPE en TPE geven bijvoorbeeld hetzelfde fluorescentiespectrum, dezelfde QY en dezelfde levensduur.
Bepaling van de levensvatbaarheid van bacteriën na blootstelling aan laser [28]
CFU-telmethode
Bacteriën (OD600 ~ 0.05) is toegevoegd met materiaal (3 of 6 μg mL −1 ) door gedurende 3 uur bij 37 °C in het donker te incuberen. Na incubatie werd het mengsel gecentrifugeerd en werden de bacteriepellets verdund (OD600 ~ 0.05) en blootgesteld aan een TPE-vermogen van 211,2 nJ pixel −1 met 800 scans (ongeveer 3.2621 s van de totale effectieve belichtingstijd; Ex, 760 nm). Dan een verdunningsfactor van 10 −5 tot 10 −8 werd vervolgens uitgevoerd in de geïncubeerde bacteriën en uitgeplaat op de agarplaten. De platen blijven een nacht in een incubator (bij 37°C). Het aantal overlevende bacteriën werd bepaald en uitgedrukt als een percentage (%) dat overeenkwam met de eenheid KVE ml −1 na incubatie. Gegevens zijn gemiddelden ± SD (n =6).
LIVE/DEAD-kit
Bacteriën (OD600 ~ 0.05) is toegevoegd met materiaal (3 of 6 μg mL −1 ) door gedurende 3 uur bij 37 °C in het donker te incuberen. Na incubatie werd het mengsel gecentrifugeerd en werden de bacteriepellets verdund (OD600 ~ 0.05) en blootgesteld aan een TPE-vermogen van 211,2 nJ pixel −1 met 800 scans (ongeveer 3.2621 s van de totale effectieve belichtingstijd; Ex, 760 nm). Vervolgens werden de pellets gekleurd met behulp van een LIVE (SYTO 9, zoals weergegeven met groene fluorescentie)/DEAD (propidiumjodide, PI, zoals weergegeven met rode fluorescentie) kit (Thermo Fisher Scientific, Waltham, MA, VS) volgens de instructie. De levensvatbaarheid van bacteriën werd gekwantificeerd voor antimicrobiële tests, waaruit bleek dat bijna alle met nanomateriaal behandelde bacteriën na behandeling dood waren. Een vergelijkbare levensvatbaarheid werd gekwantificeerd door middel van de CFU-telmethode om de efficiënte antibacteriële effecten van materialen in PDT te bepalen. Gegevens worden weergegeven als gemiddelde ± SD (n =6).
ROS-detectie [23, 29, 34, 35, 49,50,51,52,53,54,55]
Singlet zuurstof ( 1 O2 )
(a) Materiaal (3 of 6 μg mL −1 ) werd behandeld met bacteriën (OD600 ~~0,05), waarna het werd onderworpen aan een incubatietijd van 3 uur bij 37 °C in het donker. Vervolgens werd het mengsel blootgesteld aan TPE-foto-excitatie (211,2 nJ pixel −1 , 800 scans; Ex, 760 nm) en tenslotte gemengd met Singlet Oxygen Sensor Green (SOSG) reagens (1 M; Thermo Fisher Scientific, Waltham, MA, VS) (Ex/Em:488/525 nm). Voor de metingen werd een fluorescentiespectrometer gebruikt. Voor ROS-neutralisatie werd het mengsel gemengd met 30 ppm antioxidant α -tocoferol/methyllinoleaat (Sigma-Aldrich, St. Louis, MO, VS) in het donker en blootgesteld aan TPE-foto-excitatie met dezelfde behandeling. (b) Materiaal (3 of 6 μg mL −1 ) werd behandeld met bacteriën (OD600 ~~0,05), waarna het werd onderworpen aan een incubatietijd van 3 uur bij 37 °C in het donker. Vervolgens werd het mengsel blootgesteld aan TPE-foto-excitatie (211,2 nJ pixel −1 , 800 scans; Ex, 760 nm) en tenslotte gemengd met 10 μM trans-1-(2′-methoxyvinyl)pyreen (t -MVP, Thermo Fisher Scientific, Waltham, MA, VS)/0,10 M SDS (Sigma-Aldrich, St. Louis, MO, VS) (Ex/Em:352/465 nm). Voor ROS-neutralisatie werd het mengsel gemengd met 30 ppm antioxidant α -tocoferol/methyllinoleaat (Sigma-Aldrich, St. Louis, MO, VS) in het donker. Reactie van t -MVP met 1 O2 levert een dioxetaantussenproduct op dat fluoresceert terwijl het ontleedt in 1-pyreencarboxaldehyde. Bovendien reageert deze zeer selectieve fluorescerende sonde niet met andere geactiveerde zuurstofsoorten zoals hydroxylradicalen, superoxide of waterstofperoxide. Voor de metingen werd een fluorescentiespectrometer gebruikt. ROS-neutralisatie werd uitgevoerd met dezelfde als eerder beschreven behandeling.
Superoxide Radicaal Anion (O2 .− )
(a) Materiaal (3 of 6 μg mL −1 ) werd behandeld met bacteriën (OD600 ~~0,05), waarna het werd onderworpen aan een incubatietijd van 3 uur bij 37 °C in het donker. Vervolgens werd het mengsel blootgesteld aan TPE-foto-excitatie (211,2 nJ pixel −1 , 800 scans; Ex, 760 nm) en tenslotte gemengd met 2,3-bis (2-methoxy-4-nitro-5-sulfofenyl)-2H-tetrazolium-5-carboxanilide (XTT, 0,45 mM; Sigma-Aldrich, St. Louis, MO , VS). Het doel van dit materiaal was dat het in wisselwerking stond met O2 . en produceerde XTT-formazan, wat resulteerde in een sterke absorptie (470 nm in golflengte). Om deze absorptie te controleren, werd een UV-vis-spectrometer gebruikt. Voor ROS-neutralisatie werd het mengsel gemengd met 30 ppm antioxidant α -tocoferol/methyllinoleaat (Sigma-Aldrich, St. Louis, MO, VS) in het donker en blootgesteld aan TPE-foto-excitatie met dezelfde behandeling. (b) Materiaal (3 of 6 μg mL −1 ) werd behandeld met bacteriën (OD600 ~~0,05), waarna het werd onderworpen aan een incubatietijd van 3 uur bij 37 °C in het donker. Vervolgens werd het mengsel blootgesteld aan TPE-foto-excitatie (211,2 nJ pixel −1 , 800 scans; Ex, 760 nm) en tenslotte gemengd met 50 mM bicarbonaatbuffer (pH 8,60) en glutathion (γ -l-glutamyl-l-cysteinyl-glycine, GSH, Sigma-Aldrich, St. Louis, MO, VS) / 0,80 mM bicarbonaatbuffer (de Ellman's test voor O2 . detectie). Vervolgens werden de volgende experimenten uitgevoerd volgens de procedure in een eerdere studie. Verlies van GSH (%) werd berekend als het verschil in absorptie tussen het monster en de negatieve controle gedeeld door de absorptie van de negatieve controle. Het signaal van de gegenereerde O2 . verkregen zoals beschreven in de vorige berekening. Gegevens zijn gemiddelden ± SD (n =6).
Opname-assay [35]
E. coli (OD600 ~ 0.05) werden geïncubeerd met 3 μg mL −1 materiaal. De absorptie van een hoeveelheid van 3 μg mL −1 materiaal werd geregistreerd met UV-vis-spectroscopie (Abs, ongeveer 203 nm). De materialen werden gemengd met E. coli (OD600 ~~0,05) bij 37 °C van respectievelijk het 1e uur tot het 10e uur, en gecentrifugeerd (1200 rpm) om overtollig materiaal te verwijderen en het supernatant te behouden en de absorptie ervan te meten. Het verschil in absorptie tussen het verzamelde supernatant en de oorspronkelijke materialen werd geschat, resulterend in het percentage van opname op elk tijdstip. Gegevens zijn gemiddelden ± SD (n =6).
Statistische analyse [56]
De statistische significantie was door de variantieanalyse. De p waarde werd als statistisch significant beschouwd voor alle behandelingen.
Resultaten en discussie
Karakterisatie van in water oplosbaar fullerenol
In water oplosbaar C60 (OH)46 (fullerenol), waarvan werd vastgesteld dat het circulair en monodispers was, werd gesynthetiseerd in overeenstemming met een eerdere studie [27]. De gemiddelde laterale grootte van het fullerenol was ongeveer 1,13 ± 0,04 nm, zoals bepaald met behulp van lage vergroting (Fig. 2a) en HR-TEM-afbeeldingen (Fig. 2b). Verder werd opgemerkt dat het fullerenol een gunstige kristalliniteit vertoonde samen met een goede roosterafstand, wat overeenkwam met de d -afstand van de fullerenol {1\( \overline{1} \)00} roosterranden. Deze deeltjes kunnen echter aggregaten vormen door waterstofbinding in een waterige oplossing met een pH van 7,0. De gemiddelde grootte van de gevormde aggregaten was ongeveer 130 nm, zoals bleek uit een DLS-analyse. Bovendien bleven de aggregaten gedurende 3 maanden zeer stabiel in verschillende fysiologische omgevingen, zoals een pH 7.0 waterige oplossing, 1 × fosfaatgebufferde zoutoplossing en kweekmedium (aanvullend bestand 1:tabel S1). In het UV-vis absorptiespectrum van het fullerenol werden absorptiepieken waargenomen bij ongeveer 216 en 309 nm, en deze pieken werden toegeschreven aan de π –π * overgang van aromatische C=C bindingen en de n –π * overgangen van respectievelijk de C=O-schouder. De π -elektronenovergang in het fullerenol bevatte zuurstof (Fig. 2c), zoals typisch wordt waargenomen voor waterige dispersies, waardoor de aanwezigheid van het fullerenol wordt bevestigd. Aanvullende karakteriseringen werden uitgevoerd met behulp van FTIR, XPS en massaspectrometrie om de eigenschappen van de bereide materialen te bevestigen. FTIR werd gebruikt om de blootgestelde functionele groepen van de bereide materialen te analyseren. De analyseresultaten lieten de volgende karakteristieke materiaalbanden zien:een C–O-rekband op ongeveer 1109 cm −1 (band 1), fenolische C-OH-rekband op ongeveer 1271 cm −1 (band 2), tertiaire alcoholische C=O stretchband op ongeveer 1422 cm −1 (band 3), C=C rekband op ongeveer 1674 cm −1 (band 4), C=O rekband op ongeveer 1721 cm −1 (band 5), en C–H intermoleculaire waterstofgebonden en carboxylaat O–H rekband op ongeveer 3318 cm −1 (band 6). Verder een band van CO2 interferentie geconstateerd. Deze banden onthulden blootgestelde hydroxyl- en carbonylgroepen evenals aromatische C =C-bindingen (figuur 2d). XPS werd uitgevoerd om de oppervlaktechemie van het fullerenol te onderzoeken, dat voornamelijk koolstofatomen bevat. De gedeconvolueerde C (1s) spectra van de fullerenol onthulden een niet-geoxygeneerde ring (C–C/C=C, 286,1 eV), C–O-binding (286,9 eV) en C=O-binding (288,0 eV). Bovendien was de O(1s)/C(1s)-verhouding ongeveer 35,8% (figuur 2e). Het molecuulgewicht van het fullerenol werd ook bepaald met behulp van FD-massaspectrometrie (Aanvullend bestand 1:Fig. S2); the number of hydroxyl groups (C–OH) was confirmed to be 46, which was consistent with the atomic ratios and bonding compositions of the fullerenol summarized in Fig. 2. These characterization results confirm the successful synthesis of fullerenol.
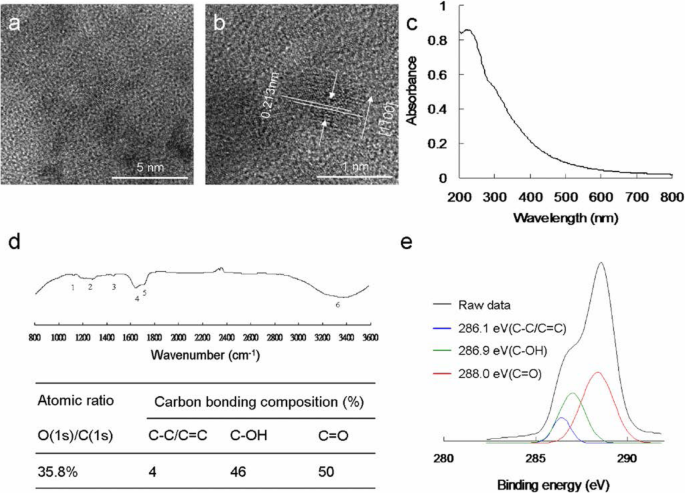
Functional characterization of the synthesized water-soluble C60 (OH)46 fullerenol. een Low-magnified TEM image and b HR-TEM image of a water-soluble fullerenol illustrating the materials {1\( \overline{1} \)00} lattice planes and the mean size of 1.11 ± 0.03 nm with a d -spacing of 0.213 nm. c UV–vis and d FTIR spectra of nanomaterial. e Deconvoluted C(1s) XPS spectra and fitted peaks obtained using Gaussian function:nonoxygenated ring (C–C/C=C), C–O bond, and C=O bond, respectively. The atomic ratio and bonding composition of fullerenol are shown as summarized in the table. The O(1s)/C(1s) atomic ratio is 35.8%
ROS Generation of Water-Soluble Fullerenol Under TPE
A PS absorbs and transfers light energy to other nonabsorbing molecules to generate ROS, which kill targeted cells, damage tumor vasculature, and activate an antitumor immune response. PSs have a particular arrangement of electrons in their molecular orbitals. Similar to nearly all molecules, at ground (singlet) state, PSs have couples of electrons with opposite spins in low-energy molecular orbitals. The absorption of light at an appropriate wavelength lifts an electron to a high-energy orbital without changing its spin. This is a short-lived (nanoseconds) excited singlet (S1 ) state, and the PS can lose its energy and return to the ground state by emitting light (fluorescence) or heat. Alternatively, intersystem crossing, wherein the spin of the excited electron is inverted, can occur in the S1 staat. This electron spin inversion is responsible for the relatively long life (lasting microseconds) of the excited triplet (T1 ) state. Radiative triplet-to-singlet transitions are inhibited because they require a change in electron spin, which is a slow process. From the T1 state, the PS can return to the ground state by emitting light (phosphorescence) or transferring energy to another molecule. It can also lose energy through internal conversion or radiationless transitions when colliding with other molecules. The longer the life of the PS in the T1 state is, the higher are its chances of colliding with another molecule, resulting in ROS production [57,58,59]. The photosensitization of water-soluble fullerenols results in their transition to a long-lived T1 state and subsequent energy or electron transfer to molecular oxygen, yielding ROS such as 1 O2 en O2 . , which have major roles in PDT. Therefore, 1 O2 en O2 . produced by water-soluble C60 (OH)46 must be detected directly using laser irradiation. To detect 1 O2 en O2 . formation during PDT, in this study, PDT was initiated by combining excited the triplet water-soluble C60 (OH)46 , oxygen, and light configured to a suitable wavelength and energy as well as by introducing SOSG, t -MVP, XTT, and GSH reagents [33, 34, 49,50,51]. To exploit the potential bactericidal capability of the materials, a wavelength of approximately 760 nm was determined to be the most efficient for deriving the relative maximum TPA ratio of the water-soluble C60 (OH)46 under TPE (Fig. 3a); this is attributable to the interband transitions involved [52]. This wavelength was used in subsequent experiments in this study. The water-soluble C60 (OH)46 was photoexcited through TPE at a power of 211.2 nJ pixel −1 with 800 scans (Ex, 760 nm; total effective exposure time, ~ 3.2621 s) and delivered dose of 3 or 6 μg mL −1 (Additional file 1:Table S2). Furthermore, to confirm the involvement of ROS in the PDT effects of the water-soluble C60 (OH)46 , α -tocopherol was used for ROS neutralization [49, 53]. The quantity of generated ROS was reduced after the addition of α -tocopherol, but the observed bacterial viability increased as expected. Additionally, the quantity of generated ROS depended on the delivered dose. To prevent 1 O2 en O2 . production possibly engendered by inadvertent exposure of water-soluble C60 (OH)46 to white light—which could have compromised the experiments in this study [60]—subsequent PDT experiments were conducted in the dark. This study focused on the quantities of generated 1 O2 en O2 . . The water-soluble C60 (OH)46 exhibited considerable antibacterial effects, demonstrating its potential for application in PDT. Notably, after the same experiment, the water-soluble C60 (OH)21 (Additional file 1:Figs. S3, S4; Fig. 3a) was less effective in forming 1 O2 en O2 . when compared with the water-soluble C60 (OH)46 (Additional file 1:Table S2). The water-soluble C60 (OH)46 generated more 1 O2 en O2 . than did the water-soluble C60 (OH)21; additionally, the water-soluble C60 (OH)46 and water-soluble C60 (OH)21 had Φ Δ values of approximately 0.93 and 0.85, respectively (for reference, Φ Δ =0.64 is the QY of TSPP dissolved in D2 O [29, 30]).
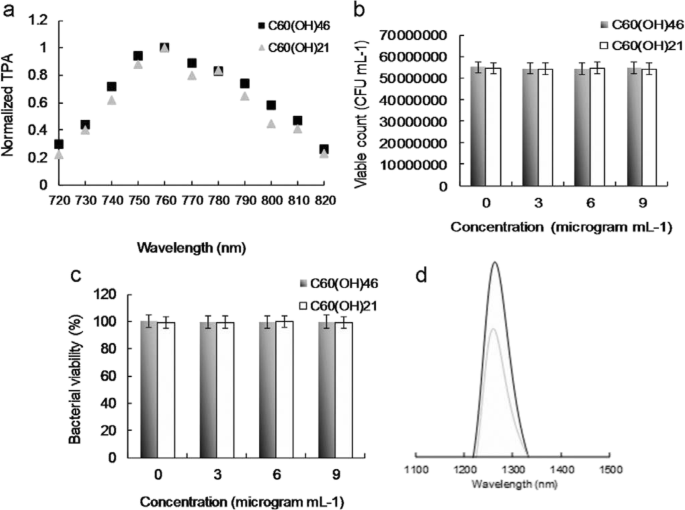
een Relative TPA spectra of the material. TPE as a function of the wavelength (720–820 nm) at 98.56 nJ pixel −1 that was used to monitor the signals. Delivered dose, 3 μg mL −1 water-soluble C60 (OH)46 or C60 (OH)21 fullerenol. The number of surviving b material-treated bacteria was determined by CFU counting assay and is expressed as the percentage (%) for c bacteria that corresponds to the unit of CFU mL −1 . Delivered dose, OD600 ~ 0.05 of E. coli and 0–9 μg mL −1 water-soluble fullerenol. d Measurement of phosphorescence spectra at 1270 nm for material. Delivered dose, 3 μg mL −1 water-soluble fullerenol. Data are means ± SD (n =6)
Antimicrobial Ability Determination Using TPE
Before the execution of antimicrobial experiments, the toxicity of water-soluble fullerenols must be examined to exclude factors that could contribute to bacterial elimination and confound experimental results. In addition, to prevent possible ROS production engendered by the inadvertent exposure of experimental materials to white light, which could confound experimental results [35], PDT experiments must be conducted in the dark. This study applied Gram-negative E. coli as the experimental template. A CFU counting assay was conducted to determine the number of surviving bacteria (expressed herein as a percentage, corresponding to CFU mL −1 ). The bacteria were treated with two types of the prepared water-soluble fullerenols (dose range, 0 to 9 μg mL −1 ) and incubated in the dark for 3 h at 37 °C to determine absorbance at 600 nm (OD600 ~ 0.05; Additional file 1:Fig. S1). The growth levels of the bacteria treated with the water-soluble fullerenols were first monitored by measuring absorbance at 600 nm. The initial absorbance was 0.05 OD600 , and the absorbance associated with both materials reached approximately 0.37 over time. Accordingly, neither material inhibited bacterial proferation. Moreover, the materials engendered a nearly 0 log10 reduction in the number of surviving bacteria (Fig. 3b), corresponding to a viability of approximately 100% (Fig. 3c). Accordingly, the materials were determined to exhibit excellent biocompatibility with the bacteria. Consequently, the materials subjected to 3 h of incubation in the dark at 37 °C were used to conduct experiments. Although the water-soluble fullerenol could generate ROS, interactions between materials and reagents (i.e., SOSG, t -MVP, XTT, and GSH) may result in false-positive ROS signals, thereby confounding PDT results [52]. Therefore, to exclude this possibility, bacteria were introduced and treated with materials in the present study. The amount of ROS generated from the photoexcited material-treated E. coli was observed. Table 2 presents the observed amount of ROS, revealing a similar trend to that in Tables S2–S3 (Additional file 1:materials alone and material-treated-Gram-positive Bacillus subtilis (B. subtilis )); these results were consistent with the 1 O2 phosphorescence signal emitted from the materials at 1270 nm (Fig. 3d). PDT against E. coli was performed using irradiation with a low dose of energy (211.2 nJ pixel −1 with 800 scans, total effective exposure time ~ 3.2621 s; Ex, 760 nm). The effects PDT on the viability of E. coli treated with two-photon photoexcited materials were then determined (Fig. 4). No bactericidal effects were observed on bacteria alone (with or without laser exposure) or on the panel of material-treated bacteria without laser treatment (Fig. 4a). After TPE, bacterial viability was relatively low; specifically, the viability observed for the panel that was treated with the water-soluble C60 (OH)21 was nearly 15%, corresponding to an approximately 0.823 log10 reduction (Fig. 4b). By contrast, the bacterial viability observed for the panel treated with the water-soluble C60 (OH)46 was approximately 0 (100% elimination efficiency, corresponding to a ~ 7.736 log10 reduction). When the dose was increased, complete bactericidal effects were observed for both materials (Fig. 4c, d). However, antimicrobial effects did not differ by bacteria type (Gram-negative E. coli or Gram-positive B. ondertiteling ) after photoexcitation (Additional file 1:Fig. S5). In addition, regarding the fullerenols that eliminated bacteria, a higher composition of hydroxyl groups increased bactericidal capability when compared with a lower composition under identical treatment conditions.
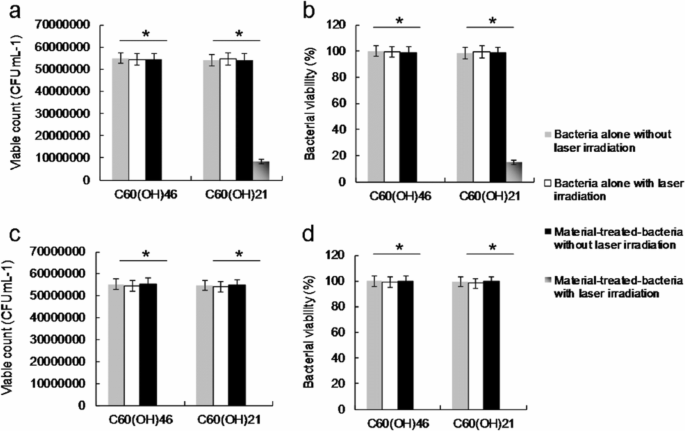
Viability (%) was quantified according to the determined viable count of material-treated bacteria through a CFU assay conducted using short excitation with a TPE power of 211.2 nJ pixel −1 with 800 scans (approximately 3.2621 s of total effective exposure time; Ex, 760 nm) to deliver a dose of a , b 3 or c , d 6 μg mL −1 . Delivered dose, OD600 0.05 of E. coli . Data are presented as means ± SD (n =6). For C60 (OH)46 - and C60 (OH)21 -treated E. coli with photoexcitation, a p <0.001 and p =0.662, b p <0.001 and p =0.658, c p <0.001 and p <0.001, and d p <0.001 and p <0.001. *p value obtained by Student’s t test
Observation of Water-Soluble Fullerenol-Treated E. coli Using TEM and Investigation of Two-Photon Properties
To observe the disruption of material-treated bacteria after photoexcitation, the water-soluble C60 (OH)46 with high PDT efficiency was selected, and bacteria were imaged using TEM. Bare E. coli (Fig. 5a) were incubated with the water-soluble fullerenol for 3 h, resulting in the substantial adsorption of materials on the bacterial surfaces. Nevertheless, no unusual morphologies were observed, indicating normal live bacterial morphology (Fig. 5b). Uptake assay results revealed the adsorption of materials onto the bacterial surface, with the corresponding burst rate being approximately 85% within the first 3 h of incubation (Fig. 5c); the rate reached saturation from the 3rd to the 10th hour. Therefore, the materials were adsorbed and formed an external barrier on the bacterial surface. However, the E. coli exhibited a distorted appearance and severe morphological changes over 3 days of incubation (Fig. 5d), resulting in a 0.940 log10 reduction that corresponded to a nearly 18% viability (Fig.5f; Additional file 1:Fig. S6). Material absorption and coating on the bacterial surface suppressed the absorption of nutrients essential for microbial growth and engendered changes in membrane (wall) permeability, thereby inducing internal osmotic imbalances and inhibiting microbial growth. In other words, the water-soluble fullerenol had antibacterial (bacteriostatic or bactericidal) effects after 3 days of incubation. Furthermore, the photoexcited material-treated bacteria, particularly E. coli , exhibited unique morphologies with severe damage after 3 h of incubation (Fig. 5e, f; Additional file 1:Fig. S6). No heat-generated bubbles formed on the bacterial surface incurred damage, indicating that the water-soluble fullerenol did not have photothermal-mediated heat properties after photoexcitation (Additional file 1:Fig. S7). The viability of E. coli was also determined through fluorescence and quantification (Fig. 6). The green fluorescence indicative of living bacteria in Fig. 6a reveals that the bacteria exposed to laser treatment alone were largely undamaged, which is consistent with the results presented in Fig. 5a. Dead bacteria were detectable after treatment with the materials and laser exposure (red fluorescence in Fig. 6b), a finding that is also consistent with that in Fig. 5e. Bacterial viability was quantified for further antimicrobial testing. Nearly complete elimination of the material-treated bacteria (Fig. 6c) was observed. Viability was also quantified using a CFU assay (Figs. 4a, b and 5f, and Additional file 1:Fig. S6) to demonstrate the antibacterial efficiency of the water-soluble C60 (OH)46 in PDT. According to the results in Figs. 4, 5, and 6; Table 2; and Table S2 (Additional file 1), E. coli treated with the water-soluble C60 (OH)46 was susceptible to photoexcitation, leading to a higher death rate, increased ROS generation, and more severe morphological collapse compared with E. coli treated with the water-soluble C60 (OH)21 . In general, the absolute cross section for TPE makes fluorophores efficient for nonlinear microscopic studies because the ratio of the energy absorbed to the input energy flux to a specimen is high, thereby minimizing possible photodamage to specimens [39, 40]. When two-photon techniques are used to image molecular activities in living biological preparations and turbid tissues, a favorable cross section is desirable [61]. In the present study, the absolute cross section for TPE calculated for the water-soluble C60 (OH)46 was approximately 1037 GM (Goeppert-Mayer unit, with 1 GM =10 −50 cm 4 s photon −1 ) at a 760-nm excitation wavelength (fluorescein was the standard reference for the cross section [39, 40]; Fig. 1b and Tables 1 and 3); the absolute cross section calculated for the water-soluble C60 (OH)21 was approximately 1230 GM, which is similar to values obtained in relevant studies [62, 63]. These absolute cross sections could facilitate the two-photon process. Moreover, the fluorescence of the water-soluble C60 (OH)46 was illuminated through a two-photon process (Fig. 1b). The relative fluorescence QY was approximately 0.02 (the QY of Cy5.5 in dimethyl sulfoxide [31] served as a reference:QYref =0.28); similarly, the absolute QY [64] was approximately 0.01, and the same QYs were derived for one-photon excitation and TPE [31]. By contrast, the water-soluble C60 (OH)21 had lower relative and absolute QYs (0.06 and 0.05, respectively). In addition, this study investigated the lifetime of the fullerenols. The effects of radiative and nonradiative decay rates on QY and lifetime were calculated. The average lifetime of the water-soluble C60 (OH)46 was approximately 7.797 ns, as calculated from observed lifetimes of 0.149, 1.775, and 19.679 ns; the average lifetime of the water-soluble C60 (OH)21 was approximately 5.251 ns (Fig. 7 and Table 4). Therefore, the ratio of radiative to nonradiative decay rates of the water-soluble C60 (OH)46 was approximately0.020 (derived from rates of approximately 2.565 × 10 6 s −1 to 1.257 × 10 8 s −1 ), whereas that of the water-soluble C60 (OH)21 was approximately 0.064 (approximately 1.143 × 10 7 s −1 and 1.790 × 10 8 s −1 ; Additional file 1:Table S4). This finding is attributable to the existence of a hydroxyl group on the surface of the water-soluble fullerenol, which induced the nonradiative recombination of electron–hole pairs, leading to the inhibition of intrinsic state emission. However, hydroxylgroups at the edge of the water-soluble fullerenol may have a high occupied molecular orbital. This can be attributed to the strong orbital interaction between hydroxyl groups, which could thus increase the efficiency of intersystem crossing (rather than fluorescence generation) and generate numerous nanomaterial triplets with a high composition of hydroxyl groups; therefore, this would result in a high Φ Δ value for the water-soluble fullerenol and induce the fullerenol to react with oxygen according to the Jablonski diagram [65]. Consequently, two-photon PDT can be effectively performed using ultralow energy in an extremely short time, thereby providing an alternative approach to killing malignant species.
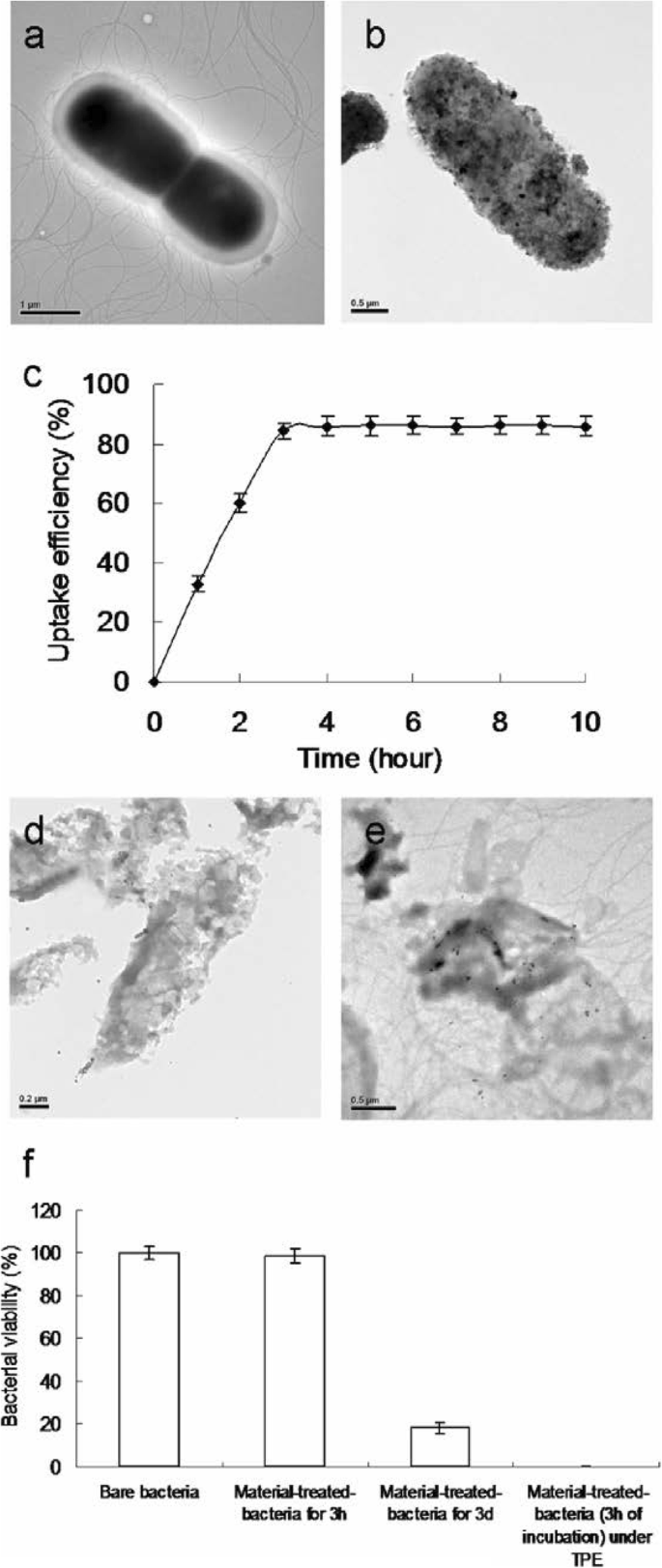
TEM-afbeeldingen. een Showing bare bacteria without any treatment. Bacteria treated with material for b 3 h and d 3 days of incubation. e The photoexcited material-treated bacteria (3 h of incubation) with a TPE power of 211.2 nJ pixel −1 with 800 scans (approximately 3.2621 s of total effective exposure time; Ex, 760 nm). c Uptake assay of bacteria and material at 37 °C. v Viability (%) was quantified following the determined viable count of material-treated bacteria via CFU assay by short excitation with the same treatment. Delivered dose OD600 ~ 0.05 of E. coli and 3 μg mL −1 water-soluble fullerenol C60 (OH)46 . Data are means ± SD (n =6)
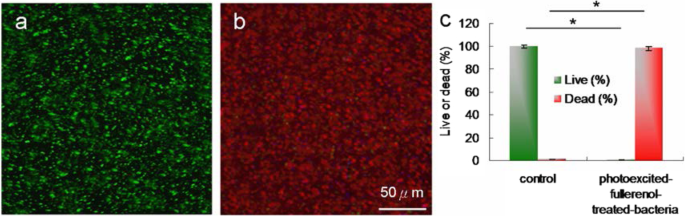
Images obtained after laser photoexcitation exposure (211.2 nJ pixel −1 ) with 800 scans (approximately 3.2621 s of total effective exposure time; Ex, 760 nm) of a , b material-treated bacteria. The Live/Dead kit was used to stain bacteria before images were obtained. Scale bar, 50 μm. c Viability (%) determination results. Delivered dose, OD600 ~ 0.05 of E. coli and 3 μg mL −1 water-soluble fullerenol C60 (OH)46 . For the percentages alive and dead, p <0.001. *p value obtained using Student’s t test. Data are presented as mean ± SD (n =6)
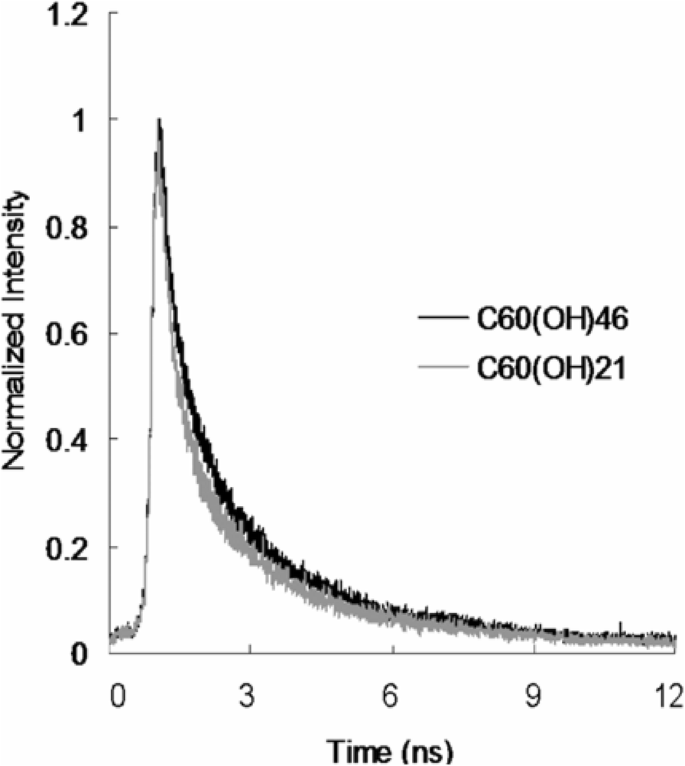
Time-resolved room-temperature PL decay profiles of material (98.56 nJ pixel −1 ). Excitation wavelength, 760 nm. Delivered dose, OD600 ~ 0.05 of E. coli and 3 μg mL −1 materialen. Data are presented as means ± SD (n =6)
Conclusions
This study revealed that a water-soluble fullerenol material with a higher composition of hydroxyl groups had superior photoproperties to those of a fullerenol material with a lower composition of hydroxyl groups; the superior photoproperties can be attributed to the reduced laser exposure and materials used for treatment. Furthermore, the water-soluble fullerenol with a higher composition of hydroxyl groups exhibited high TPA, a favorable absolute cross section for TPE, and high two-photon stability. Therefore, this fullerenol has potential as a two-photon PS in two-photon PDT coupled with TPE. This property is probably due to the presence of a hydroxyl group on the surface of the water-soluble fullerenol, which caused the nonradiative recombination of electron–hole pairs, leading to the inhibition of intrinsic state emission. Moreover, hydroxyl groups at the edge of the water-soluble fullerenol may have a high occupied molecular orbital; this may be ascribed to the strong orbital interaction between the hydroxyl groups, thereby increasing intersystem crossing (rather than fluorescence generation) efficiency and generating numerous material triplets with a high composition of hydroxyl groups. Therefore, the water-soluble fullerenol would have a high Φ Δ value and react with oxygen according to the Jablonski diagram. Consequently, two-photon PDT can be effectively performed using ultralow energy in an extremely short time. Accordingly, this efficient alternative approach to managing malignant species presents possibilities for future clinical applications.
Beschikbaarheid van gegevens en materialen
All datasets are presented in the main paper.
Afkortingen
- PS:
-
Photosensitizers
- QY:
-
Kwantumopbrengst
- PDT:
-
Fotodynamische therapie
- UV–vis:
-
Ultraviolet zichtbaar
- ROS:
-
Reactieve zuurstofsoorten
- ψ Δ :
-
Singlet oxygen QY
- NIR:
-
Nabij-infrarood
- TPE:
-
Two-photon excitation
- E. coli :
-
Escherichia coli
- HR-TEM:
-
Transmissie-elektronenmicroscopie met hoge resolutie
- DLS:
-
Dynamische lichtverstrooiing
- FTIR:
-
Fourier-transform infrarood
- XPS:
-
Röntgenfoto-elektronenspectroscopie
- FD:
-
Field desorption
- CFU:
-
Colony forming unit
- TSPP:
-
Meso -tetra(4-sulfonatophenyl)porphine dihydrochloride
- PL:
-
Fotoluminescentie
- DMSO:
-
Dimethylsulfoxide
- OPE:
-
One-photon excitation
- TPA:
-
Two-photon absorption
- TPL:
-
Two-photon luminescence
- ti-sa:
-
Titanium-sapphire
- NA :
-
Numerical aperture
- FLIM:
-
Fluorescence lifetime imaging microscopy
- 1 O2 :
-
Singlet oxygen
- SOSG:
-
Singlet Oxygen Sensor Green
- t -MVP:
-
Trans-1-(2′-methoxyvinyl)pyrene
- O2 . :
-
Superoxide radical anion
- XTT:
-
2, 3-Bis (2-methoxy-4-nitro-5-sulfophenyl)-2H-tetrazolium-5-carboxanilide
- GSH:
-
Glutathione, γ -l-glutamyl-l-cysteinyl-glycine
- S1 :
-
Singlet
- T1 :
-
Triplet
- B. ondertiteling :
-
Bacillus subtilis
- GM:
-
Goeppert-Mayer
Nanomaterialen
- MoS2 met gecontroleerde dikte voor elektrokatalytische waterstofevolutie
- S, N co-gedoteerde grafeen Quantum Dot/TiO2-composieten voor efficiënte fotokatalytische waterstofgeneratie
- Elektrospun polymeer nanovezels versierd met edele metalen nanodeeltjes voor chemische detectie
- Monodispergerende koolstofnanosferen met hiërarchische poreuze structuur als elektrodemateriaal voor supercondensator
- Verkenning van Zr–Metal–Organic Framework als efficiënte fotokatalysator voor waterstofproductie
- Hiërarchische heterostructuur van ZnO@TiO2 holle bollen voor zeer efficiënte fotokatalytische waterstofevolutie
- Een efficiënt en effectief ontwerp van InP-nanodraden voor maximale oogst van zonne-energie
- S-gedopeerd Sb2O3-nanokristal:een efficiënte zichtbaar-lichtkatalysator voor organische afbraak
- Vervaardiging van 20,19% efficiënte enkelkristallijne siliciumzonnecel met omgekeerde piramidemicrostructuur
- Fox Chase werkt samen met de Rodon Group om COVID-teststaafje voor tempelgezondheid te ontwikkelen
- Volvo Group werkt samen met Nvidia op AI-platform voor autonome vrachtwagens