Een gemakkelijke eenpotsynthese van biomimetische fotokatalysator Zn(II)-porfyrine-gevoelige 3D TiO2-holle nanoboxen en synergetisch verbeterde degradatie van zichtbaar licht
Abstract
Een serie biomimetische fotokatalysator zink(II) meso -tetra(4-carboxyfenyl)porfyrinato (ZnTCP)-gesensibiliseerde 3D hiërarchische TiO2 holle nanoboxen (TiO2 -HNB's) samengesteld door zes geordende nanosheets met dominante {001} facettenblootstelling (ZnTCP@TiO2 -HNB's) zijn met succes gesynthetiseerd door een gemakkelijke eenpotssolvotherme methode via een topologisch transformatieproces met TiOF2 als sjabloon. Infraroodspectra (IR), UV-vis-spectroscopie en röntgenfoto-elektronspectroscopie (XPS) bevestigden dat ZnTCP een beslissende rol speelde bij het construeren van 3D holle nanoboxen door de vorming van een esterbinding gecombineerd met TiO2 -HNB's, die ook een overdragende foto-geëxciteerde elektronenbrug leverden om TiO2 te sensibiliseren -HNB's voor het verbeteren van de respons op zichtbaar licht. Vanwege de superieure sensibilisatie en biomimetische activiteit van ZnTCP, is de fotodegradatiesnelheid van rhodamine B (RhB) van zoals bereid ZnTCP@TiO2 -HNB's met ZnTCP/TiOF2 massaverhouding van 2% (T-2p) verbetert 3,6 keer vergeleken met die van TiO2 -HNB's met een afbraakrendement van 99% gedurende 2 uur onder gesimuleerde zonlichtinstraling (> 420 nm). Het verbeterde fotodegradatievermogen werd toegeschreven aan het synergetische zichtbare fotokatalytische mechanisme van de biomimetische katalysator, die niet alleen hydroxylradicaal (•OH) en superoxideradicaal (•O2 kan produceren) − ) afkomstig van het excitatieproces van met ZnTCP gesensibiliseerde TiO2 -HNB's, maar genereren ook singlet-zuurstof ( 1 O2 ) die alleen werd geleverd door het biomimetische enzym porfyrines. Verder vertoonde de fotokatalysator een goede recyclingstabiliteit en dispergeerbaarheid na vijf ronden, toegeschreven aan ZnTCP sterke chemische binding aan de drager TiO2 -HNB's. Door middel van elektrochemische cyclische voltammetrie-analyse werd het effect van centrale zinkionen en ouderporfyrineringen op de redoxeigenschap van biomimetische katalysatoren bestudeerd.
Achtergrond
Allerlei organische kleurstoffen spelen een niet-vervangbare rol in verschillende industriële toepassingsgebieden, waaronder het verven van textiel, leer of papier [1]. Hun toxiciteit, diversiteit en persistentie hebben echter een directe invloed op de gezondheid van ecosystemen en vormen een directe bedreiging voor de mens door verontreinigde drinkwatervoorraden [2]. Het wordt erkend als een dringende uitdaging om een eenvoudige manier te vinden om synthetische kleurstofverontreinigende stoffen uit industrieel afvalwater te verwijderen.
Er zijn talloze methoden ontwikkeld om de verwijdering van organische kleurstoffen uit afvalwater aan te pakken, waaronder fysische, biologische en chemische methoden [3,4,5]. De fysieke methoden zoals adsorptie, coagulatie, ionenuitwisseling en membraanfiltratie worden gebruikt om organische verontreinigende stoffen te verwijderen [6, 7], terwijl fysieke methoden alleen de organische moleculen weghalen van de waterfase en het probleem niet fundamenteel oplossen. Bovendien vinden biologische processen meestal plaats onder relatief milde omstandigheden, vanwege hun inherente tijdrovende procedure en hun intolerantie voor organische verontreinigende stoffen, wat het moeilijk maakt voor grootschalige industriële toepassingen. Omdat het gebruik maakte van zonne-energie voor de afbraak van kleurstofverontreinigende stoffen, vormde de gevormde AuAgCuBi2 O4 composiet vertoont een uitstekende fotokatalytische activiteit tegen de afbraak van RhB onder gesimuleerde zonlichtbestraling. En de composieten vertonen een significant verhoogde fotokatalytische activiteit [8,9,10,11]. Op basis van bovenstaande overwegingen is de effectieve verwijdering van organische verontreinigende stoffen uit afvalwater verre van bevredigend, en er is nog steeds een dringende noodzaak om kosteneffectieve en milieuvriendelijke strategieën te ontwikkelen. Geavanceerde oxidatieprocessen (AOP's) op basis van de productie van actieve soorten, zoals hydroxylvrije radicalen, die het probleem van kleurstofvernietiging in waterige systemen aankunnen, zijn gebruikt om selectieve oxidatie van een breed scala aan organische verontreinigende stoffen te voorkomen [12] . Enkele typische AOP's-technieken zijn met succes ontwikkeld voor de behandeling van organische verontreinigende stoffen, waaronder Fenton-schema's, ozonsystemen, halfgeleiderfotokatalysatoren en elektrochemische katalyse. Vanwege de eenvoudige werking en milieuvriendelijkheid is er de afgelopen jaren veel aandacht besteed aan de afbraak van organische verontreinigende stoffen gekatalyseerd door biomimetische katalyse, die de voordelen van de herkenning van enzymen en chemische katalyse samenbrengt [13].
Vergeleken met chemische katalyse is de hoge selectiviteit een van de grootste voordelen van biomimetische katalyse, en het kan worden gebruikt onder milde en milieuvriendelijke omstandigheden, wat de energie-input en afvalproductie aanzienlijk kan verminderen. Onlangs hebben de metalloporfyrine en zijn derivaten, die het oxidatiekarakteristieke proces van peroxidase-enzymen of ijzerbevattende oxygenase kunnen nabootsen, veel aandacht getrokken voor gebruik als oxidatieve afbraak van persistente organische verontreinigende stoffen vanwege de goede chemische stabiliteit en hun hoge absorptiecoëfficiënt in de zonnestraling. spectrum. Zhao meldde dat ijzer (II) bipyridine, gedragen op een ion-uitgewisselde hars, O2 effectief zou kunnen activeren om organische kleurstoffen in water af te breken onder bestraling met zichtbaar licht [14]. Collins synthetiseerde een reeks ijzer-gecentreerde tetraamido macrocyclische ligandcomplexen, die in staat waren om zowel H2 O2 en O2 om organische verontreinigingen in heterogene waterige systemen af te breken om peroxidase-achtige processen met enzymatische snelheden na te bootsen. Onze groepen rapporteerden dat ijzertetra (1,4-dithiin) porfyrazine gedragen op anionenuitwisselingshars (amberliet CG-400) uitstekende oxidatieve afbraak van organische kleurstoffen vertoonde. Rekening houdend met de gerapporteerde, is gebleken dat deze metalloporfyrines en zijn derivaten allemaal gebaseerd zijn op het centrale ijzerion, vanwege de variabele valentie van ijzerion. Echter, omdat Fe 2+ is veel moeilijker te chelateren in de porfyrinering en atmosferische instabiliteit, dit proces wordt meestal uitgevoerd onder zware omstandigheden en lange reactietijden.
Zoals we allemaal weten, hebben zinkporfyrines en zijn metalloporfyrine aantrekkelijke eigenschappen vertoond, zoals hoge stabiliteit, goede lichtgevoeligheid, niet-toxische en gemakkelijke synthese, die door steeds meer wetenschappers worden begunstigd. Met name de fotosensitiviteit van zinkporfyrines kan de kwantumopbrengst van singletzuurstof induceren om te gebruiken voor fotodynamische therapie en fotokatalytische oxidatieve afbraak van organische verontreinigende stoffen [15]. Deze uitstekende eigenschappen inspireren ons om betere manieren te vinden voor het ontwerpen van gemakkelijke synthese van zinkporfyrinecomposieten om de fotokatalytische oxidatie van organische verontreinigende stoffen te bestuderen. Tegelijkertijd werden, gezien de hydrofiliciteit van zinkporfyrine, vier carboxylgroepen geïntroduceerd in de perifere grote ring.
Een ander dringend probleem was nodig om de dragers van zinkporfyrinecomposieten op te lossen. In overeenstemming met de eerdere rapporten van onze groep, vertonen metalloporfyrinen en metalloporfyrazinen alleen uitstekende heterogene fotokatalytische activiteit wanneer ze worden ondersteund op anionenuitwisselingshars (Amberlite CG-400) of ionenuitwisselingshars. Alle soorten harsen kunnen echter geen elektronen- en gatenparen genereren en de ladingen snel overdragen onder bestraling met zichtbaar licht. Daarom richten we onze ogen op een typische halfgeleider fotokatalysator TiO2 .
Tot nu toe, anatase TiO2 is een van de belangrijkste fotokatalyse geworden vanwege zijn potentiële toepassing bij het oplossen van milieuvervuiling en energiecrisis, zoals fotokatalytische afbraak van organische verontreinigende stoffen in afvalwater vanwege zijn niet-toxiciteit, hogere chemische stabiliteit en fotostabiliteit. Het kan echter slechts een zeer klein ultraviolet deel (ongeveer 4,5%) van het zonlicht absorberen vanwege de relatief brede bandgap van anatase TiO2 (3.2 eV), wat de praktische toepassing ervan ernstig beperkt [16,17,18]. Daarom is het van groot belang voor het verbeteren van de fotoreactie-activiteit van TiO2 in zichtbaar licht. -gebaseerde fotokatalysatoren om de overvloedige hoeveelheid van het zonnespectrum volledig te benutten, maar blijft een grote uitdaging. Tot op heden is er een aanzienlijke hoeveelheid studies gewijd aan het verbeteren van het gebruik van TiO2 in zichtbaar licht. door halfgeleiderkoppeling [19,20,21], dotering van metalen en niet-metaalionen [22, 23] en oppervlaktegevoelige modificatie [24,25,26]. Dit kan worden verklaard door het feit dat de zuurstofvacatures aan het oppervlak fungeren als foto-geïnduceerde ladingsacceptoren en adsorptieplaatsen de recombinatie van fotogegenereerde ladingen onderdrukken, wat leidt tot een toenemende beschikbaarheid van fotogegenereerde elektronen en gaten voor fotokatalytische reactie [27,28,29]. Met betrekking tot halfgeleiderkoppeling, hoewel het de scheidingssnelheid van fotogegenereerde elektron-gatparen effectief kan verhogen, hangt hun fotoreactie-efficiëntie sterk af van hun junctiestructuren en verkregen ze alleen een te lage energieconversie-efficiëntie voor industriële toepassing [30,31,32]. De dopingmodificatie van TiO2 is over het algemeen nogal moeilijk te bereiden met roosteruitwisseling bij hoge temperatuur en meerstapsprocedures. De oppervlaktesensibilisatiebenadering kan het lichtgevoelige gebied van TiO2 . drastisch uitbreiden van UV tot zichtbaar licht bereik. Als een goede sensibilisator, metalloporfyrine en zijn derivaat-gesensibiliseerde TiO2 hebben veel aandacht getrokken vanwege hun hoge absorptiecoëfficiënt binnen de zonnespectra, kleine singlet-triplet-splitsing, hoge kwantumopbrengst voor intersysteemkruising, de lange levensduur van triplettoestanden en goede chemische stabiliteit [33, 34]. Wat nog belangrijker is, is dat sensibilisatie voor porfyrine de bandgap van TiO2 kan vergroten. optische absorptie in het gebied van zichtbaar licht, die de afbraak van organische verontreinigende stoffen efficiënt kan katalyseren. De functionele groepen rond de porfyrinering zijn het kanaal dat ervoor zorgt dat de elektronen in de aangeslagen toestand van de kleurstof worden overgebracht naar TiO2 geleidingsband [35, 36].
Onlangs is een grote hoeveelheid 3D TiO2 holle nanostructuren zijn gesynthetiseerd door allerlei methoden, zoals 3D holle nanobuisjes of microsferen opgebouwd uit nanodraden, nanosheets, nanorods of nanodeeltjes. Deze holle structuren, bestaande uit willekeurig samengestelde bouwstenen, zijn echter voornamelijk gebaseerd op een bolvormige structuur. Het streven naar niet-sferische 3D TiO2 holle nanomaterialen, zoals een doosachtige of kubusachtige structuur, samengesteld uit een geordende hiërarchische structuur, blijft nog steeds een uitdaging. Tot op heden heeft zowel experimentele als theoretische analyse aangetoond dat de hoge energie {001} facetten van anatase TiO2 kan fotogegenereerde elektron-gatparen efficiënt scheiden en de bandgap van TiO2 . verkleinen , die een explosie van onderzoeksinteresse heeft gewekt [37]. Op basis van de bovenstaande beschouwing van morfologie en facetmodificatie, hoe 3D hiërarchische TiO2 te construeren holle doosachtige structuren, samengesteld door zes geordende geordende vellen, met dominante belichting van {001} facetten om de absorptie van zichtbaar licht te verbeteren, blijft nog steeds een moeilijk probleem. Er is dus een dringende vraag naar de ontwikkeling van een gemakkelijke en één-pot topologische synthetische strategie om porfyrine-gesensibiliseerde 3D holle TiO2 te synthetiseren. structuren om een door zichtbaar licht gestuurde respons te verkrijgen. Voor zover wij weten, is de in situ fluoride-geïnduceerde zelftransformatie kubische TiOF2 voor de bereiding van holle nanoboxen van zinkporfyrine-gesensibiliseerd TiO2 samengestelde fotokatalysator door solvothermische behandeling is niet gerapporteerd.
Hierin hebben we meso . voorgesteld -tetra (4-carboxyfenyl) porfyrinatozink (II) (ZnTCP) met carboxylgroep op porfyrineperiferie als sensibilisator en gebruikte een gemakkelijke eenpots topologische solvotherme methode om ZnTCP-gesensibiliseerde TiO2 te bereiden holle nanoboxen (ZnTCP@TiO2 -HNB's) met dominante {001} facettenblootstelling door de vorming van stabiele covalente bindingen tussen TiO2 -HNB's en zinkporfyrinen. We hebben systematisch het effect bestudeerd van het gehalte aan zinkporfyrinemassa op de structuur en zichtbare fotokatalytische activiteit van met zinkporfyrine gemodificeerd TiO2 holle nanoboxen (ZnTCP@TiO2 -HNB's).
Methoden
Materialen
Alle reagentia en oplosmiddelen waren van reagenskwaliteit, verkregen van commerciële leveranciers (Wuhan Guoyao Chemical Reagent Co. Ltd.) en direct gebruikt zonder verdere zuivering. Alle oplossingen werden bereid met gedestilleerd water.
Synthese van ZnTCP-gesensibiliseerde TiO2 -HNB's (ZnTCP@TiO2 -HNB's)
TiOF2 Precursor werd eerst voorbereid als de nanocubic-sjabloon
In een typische procedure werden 30 ml acetaat, 5 ml fluorwaterstof en 15 ml tetrabutyltitanaat druppelsgewijs toegevoegd aan een bekerglas van 100 ml polyvinylfluoride en continu 25 minuten geroerd. Vervolgens werd het mengsel overgebracht naar een gedroogde 100 ml met Teflon beklede autoclaaf en gedurende 12 uur op 200°C gehouden. Na te zijn afgekoeld tot kamertemperatuur, werd het witte poeder verschillende keren gewassen met ethanol en een nacht onder vacuüm gedroogd bij 60°C, waarna TiOF2 verkregen werd. nanocubic voorloper.
Bereiding van Zn Porphyrins (ZnTCP)
De voorbereidingsprocedure omvatte twee stappen. De eerste stap is de synthese van porfyrine (TCP):doorgaans werden 100 ml propionzuur en 4,341 g (28,8 mmol) 4-carboxybenzaldehyde toegevoegd aan een driehalskolf van 500 ml, die in een oliebad werd verwarmd en gedurende 30 minuten werd geroerd. volledige verspreiding te garanderen. Een oplossing van 10 ml propionzuur en 2 ml pyrrool werd langzaam druppelsgewijs toegevoegd aan het bovenstaande systeem en gedurende 2 uur bij 140 °C onder terugvloeikoeling gekookt. Het mengsel werd afgekoeld tot kamertemperatuur en tweemaal gewassen met 150 ml heet water. Gebruik vervolgens natriumcarbonaat en zwavelzuur om teer te verwijderen en de pH-waarde in te stellen op respectievelijk 5 tot 7. Het product werd driemaal geëxtraheerd met butanol; tenslotte werd het product geconcentreerd en gedroogd door middel van roterende verdamping. 1 H NMR-spectrum van TCP toonde de verwachte signalen zoals -OH, Ar-H (fenyl) en -CH (pyrrool) protonen bij respectievelijk 4,12, 6,98-7,02 en 7,46-7,57 ppm. NH-protonen in de binnenste kern verschijnen als een door deuterium uitwisselbare brede chemische verschuiving bij = − 3.34 ppm als gevolg van de 18 π-elektronensystemen van de vlakke structuur. IR (KBr, cm −1 ) spectra, 3363 voor -OH, 2927, 2852 voor C-H, 1712 (C=O), 1623, 1488 en 1376 voor C=C, 1053 voor COOH, bevestigt de TCP-structuur. Het massaspectrum vertoonde een moleculaire ionpiek bij m/z = 614.14 [M + 1] + . UV-vis [DMF, max/nm] B-band bij 419 nm en Q-band bij 553 en 598 nm.
Ten tweede was de synthese van ZnTCP als volgt:0,544 g porfyrinen en 0,20 g zinkacetaat werden opgelost in DMF-oplosmiddel. De mengseloplossing werd onder continu roeren 2 uur verwarmd en onder terugvloeikoeling gekookt tot 150°C onder gebruikmaking van een oliebad. Daarna werd het afgekoeld tot kamertemperatuur, het product werd geconcentreerd en er werd 100 ml gedeïoniseerd water toegevoegd nadat de paarse precipitatie volledig was neergeslagen. Ten slotte werd het water verwijderd door roterende verdamping en meso -tetra (4-carboxyfenyl)porfyrinezink (II) (ZnTCP) werd verkregen. In de 1 H NMR-spectra van ZnTCP, NH-protonen in de binnenste kern verdwenen; andere protonen vertoonden de vergelijkbare chemische verschuiving bij 4,12, 6,98-7,02 en 7,46-7,57 ppm. Uit het IR-spectrum, de scherpe piek van C=O-bindingstrillingen rond 1720 cm −1 was versterkt na de cyclotetramerisatiereactie, 3352 cm −1 voor -OH, 2928, 2853 cm −1 voor C-H, 1619, 1504 cm −1 en 1378 cm − 1 voor C=C, 1231 en 1017 cm −1 voor C=O, bevestigde de structuur van ZnTCP. De massaspectrumgegevens van ZnTCP bevatten een sterke piek bij m/z = 678.11 [M + 1] + voor het ouderringion. Het XPS-spectrum bewees de aanwezigheid van 2p1/2 Zn(II)-pieken met bindingsenergie van 1021,5 eV en 2p3/2 met bindingsenergie van 1045,6 eV. UV-vis [DMF, max/nm] B-band bij 432 nm en Q-band bij 561 en 600 nm. De synthetische procedure van ZnTCP wordt geïllustreerd in Schema 1.
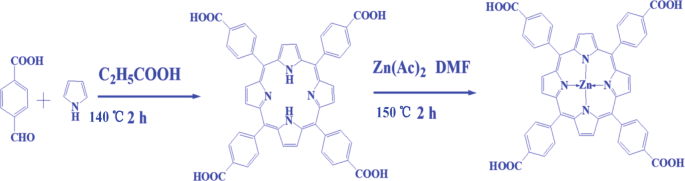
Schematische illustratie van de synthese van het ZnTCP-complex
Een gemakkelijke éénpot gesynthetiseerd van ZnTCP-gevoelig TiO2 -HNB's (ZnTCP@TiO2 -HNB's)
TiOF2 gebruiken als template, ethanol als oplosmiddel en zinkporfyrines als sensibilisator en TiO2 holle nanoboxen als dragers, met porfyrines gesensibiliseerde 3D hiërarchische titaandioxide holle nanoboxen met blootgestelde hoge energie {001} facetten werden bereid via een topologisch transformatieproces. In een typische procedure 300 mg TiOF2 , 70 ml ethanol en 3 mg ZnTCP werden in met Teflon beklede autoclaaf geplaatst en gedurende 48 uur bij 200 ° C onder reactie geplaatst, waarna het werd afgekoeld tot kamertemperatuur; het product werd geconcentreerd door rotatieverdamping en een nacht onder vacuüm gedroogd bij 60°C. Vervolgens werd het verkregen product aangeduid als T-1p, waarbij 1 staat voor ZnTCP/TiOF2 massa verhouding. Ter vergelijking werden ook verschillende monsters gesynthetiseerd onder andere identieke omstandigheden, behalve de hoeveelheid zinkporfyrines. Ze worden respectievelijk aangeduid als T-0p, T-1p, T-2p, T-3p en T-5p. Het één-pots synthetische proces van ZnTCP@TiO2 -HNB's worden weergegeven in Schema 2.
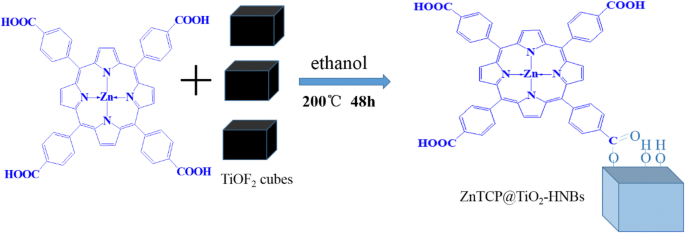
Schematische illustratie van de bereiding van ZnTCP@TiO2 -HNB's
Karakterisering
Fourier-transformatie-infraroodspectroscopie (FT-IR) werd gemeten op een NeXUS 470-spectrometer met behulp van KBr-pellettechniek. De kristallijne structuur van deze katalysatoren werd gekenmerkt door poederröntgendiffractie (XRD) met een scansnelheid van 0,05°s −1 in een 2θ bereik van 10 tot 80 °, in een Bruker D8 Advance met behulp van monochromatische Cu-Ka-straling. Röntgenfoto-elektronspectroscopie (XPS) werd opgenomen op een VG Multilab 2000-foto-elektronspectrometer met behulp van monochromatisch Mg Ka straling onder vacuüm bij 2 × 10 −6 Pa. Alle bindingsenergieën waren gerelateerd aan de C1s-piek bij 284,8 eV van de oppervlakte-adventieve koolstof. De morfologieën en microstructuren van voorbereide monsters werden geanalyseerd met een veldemissie scanning elektronenmicroscoop (SEM) (Hitachi, Japan) en een transmissie-elektronenmicroscoop (TEM) (Tecnai G 2 20, VS). De UV-vis diffuse reflectiespectroscopie (DRS) werd verzameld met een Shimadzu UV-2600-spectrofotometer van 240 tot 800 nm met BaSO4 als achtergrond. Fotoluminescentie (PL) spectra werden verkregen op een Hitachi F-7000 fluorescentie spectrofotometer. De metingen van de cyclische voltammetrie (CV) werden uitgevoerd op een elektrochemisch werkstation dat werd bestuurd door een externe pc en gebruikmakend van een configuratie met drie elektroden bij kamertemperatuur.
Fotokatalytische metingen
Alle fotoreactie-experimenten werden uitgevoerd in een zelfgemaakt fotokatalytisch reactorsysteem, de fotokatalytische activiteiten van voorbereide monsters werden geëvalueerd met organische kleurstoffen RhB (1 × 10 −5 mol/L) als de doelverontreinigende stof onder bestraling met zichtbaar licht. Het specifieke proces is als volgt:50 mg fotokatalysator en 50 ml beginconcentratie van 5 × 10 −4 mol/L RhB-oplossing werd toegevoegd aan een cilindrisch reactievat door middel van ultrasone dispersie met een 14 cm 2 vlakke zijde en 7 cm hoogte, en vervolgens werd de gemengde oplossing een nacht in het donker geoscilleerd. Nadat het adsorptie-evenwicht was bereikt, werd de fotokatalytische reactie gestart door het systeem te bestralen met een 210 W xenonlamp met lichtfilter (λ> 420 nm) om te zorgen voor verlichting door alleen zichtbaar licht, werd het systeem continu gekoeld met water, dat werd gebruikt om het systeem op kamertemperatuur te houden. Op bepaalde tijdsintervallen werden hoeveelheden van 3,5 ml verzameld en gecentrifugeerd en vervolgens werden de katalysatordeeltjes verwijderd voor analyse. De concentratie van RhB met verschillende tussenpozen werd gevolgd door UV-vis-spectroscopie. Alle metingen zijn uitgevoerd bij kamertemperatuur.
De fotoluminescentie (PL)-techniek werd gebruikt om de actieve soorten te bestuderen die tijdens de afbraak van de katalysator worden geproduceerd. Volgens de kostbare gerapporteerde [38], werden cumarine, 4-chloor-7-nitrobenz-2-oxa-1, 3-diazool (NBD-Cl), en 1, 3-difenylisobenzofuran (DPBF) gebruikt als fluorescerende sonde om te detecteren hydroxylradicaal (•OH), superoxideradicaal (•O2 − ), en singlet zuurstof ( 1 O2 ), respectievelijk. ZnTCP@TiO2 -HNB's katalysator en water reageren om de actieve soort hydroxylradicalen (•OH) te vormen en worden vervolgens snel gevangen door cumarine om 7-hydroxycoumarine te genereren dat een sterke fluorescentie-eigenschap heeft. Typisch is de gedetecteerde procedure als volgt:De suspensies van katalysatoren (1,0 g/L) die cumarine (0,1 mmol/L) bevatten, worden gemengd onder magnetisch roeren en werden vervolgens een nacht geschud. Het mengsel werd bestraald met 210 W xenonlamplicht en het monster werd genomen met de ingestelde intervallen van 2 minuten (of 4 minuten of 15 s). De fluorescentiespectrofotometer werd gebruikt om filtraat te analyseren door de excitatie met de relevante golflengte.
Resultaten en discussie
TEM- en SEM-afbeeldingen
De morfologie en kristalvlakken van TiOF2 , TiO2 -HNB's en ZnTCP@TiO2 -HNBs-monsters werden waargenomen door TEM geïllustreerd in Fig. 1. Figuur 1a laat zien dat TiOF2 onthult uniform solide kubieke vorm en glad oppervlak met een gemiddelde grootte van 250 nm. Zoals weergegeven in Fig. 1b, is te zien dat de morfologie van TiO2 -HNB's getransformeerd in een uniforme holle doosachtige vorm bestaande uit zes geordende vlakken met een gemiddelde zijlengte van 260 nm, wat consistent was met de kubieke TiOF2 sjabloon. Als we goed kijken naar Fig. 1c-f, kunnen we zien dat 3D holle structuren van ZnTCP@TiO2 -HNB's vertoonden nog steeds een goed gedefinieerde doosvormige morfologie tijdens de fluoride-geïnduceerde zelftransformatie kubische TiOF2 tot ZnTCP-gesensibiliseerde TiO2 holle nanoboxen-proces, die goed gevormd waren met enkele harige tentakels van ZnTCP bedekt op het oppervlak van holle dozen [39]. HRTEM-afbeeldingen (zie het inzetstuk van Fig. 1g) werden verkregen om te bevestigen dat de roosterranden ongeveer 0,235 nm waren, wat goed overeenkwam met het hoogenergetische {001} kristallietfacet van anatase TiO2 . Het oppervlak van ZnTCP@TiO2 -HNB's roosterdiagram vertoonde 3D hiërarchische holle nanoboxen bestaande uit zes geordende dominante blootgestelde {001} facetten nanosheets.
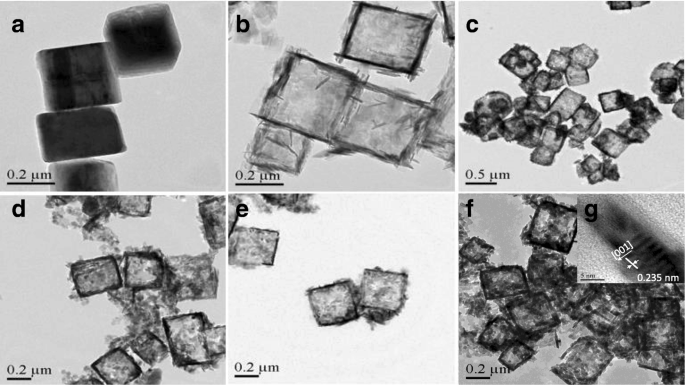
TEM-afbeeldingen van gesynthetiseerde katalysatoren:a TiOF2 , b TiO2 -HNB's, c T-1p, d T-2p, e T-3p en f , g T-5p
De oppervlaktemorfologie van TiOF2 , TiO2 -HNB's en ZnTCP@TiO2 -HNB's werden geïdentificeerd aan de hand van de SEM-afbeeldingen geïllustreerd in Fig. 2. Figuur 2a bestaat uit uniform en volledig kubisch TiOF2 in overeenstemming met de TEM-afbeeldingen. Zoals weergegeven in Fig. 2b, zijn de afbeeldingen van TiO2 -HNB's vertoonden goed gevormde morfologie van holle dozen, die is afgeleid van de sjabloon TiOF2 kubussen in de topologische transformatieprocedure en omsloten door zes geordende TiO2 nanosheets-arrays. Zoals weergegeven in Fig. 2c-f, alle ZnTCP@TiO2 -HNBs-monsters vertoonden ook volledig holle doosachtige structuren met harige tentakels van Zn (II) porfyrine die op het oppervlak van nanoboxen bedekten, wat goed consistent was met TEM-resultaten, wat het succesvolle topologische transformatieproces in één pot van kubieke TiOF impliceert 2 naar TiO2 holle nanoboxen met ZnTCP in situ deelname.
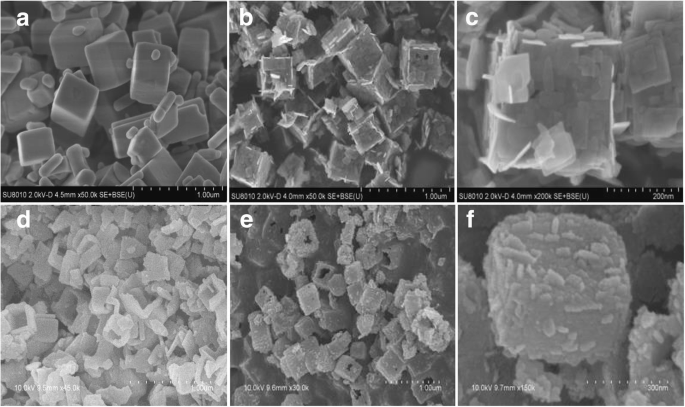
SEM-afbeeldingen van voorbereide katalysatoren:a TiOF2 , b TiO2 -HNB's, c T-1p, d T-2p, e T-3p en f T-5p
Zoals beschreven in Fig. 3, het waarschijnlijke vormingsmechanisme van Zn(II)-porfyrine-gesensibiliseerde 3D TiO2 holle nanoboxen (ZnTCP@TiO2 -HNB's) is voorgesteld via een proces van topologische transformatie waarbij sjabloonparticipatie betrokken is. Ethanol zorgde voor milde reactieomstandigheden door een oplosmiddel te gebruiken, waarvan de dehydratatiereactie (Vgl. 1, hoofd) en esterreactie tussen ZnTCP en ethanol (Vgl. 2) H2 leverden O en bevorderde de hydrolyse van kubische TiOF2 , die topologisch kan transformeren naar anataas TiO2 holle doosvormige nanokristallen tijdens solvotherme reactie (vergelijking 3). Omdat de adsorptie van F − ion op het oppervlak van TiO2 nanokristallen kunnen de oppervlakte-energie van (001) facetten sterk verminderen, fluoride-ionen vergemakkelijken de vorming van hoogenergetische anatase TiO2 nanosheets en het is begrijpelijk dat voorloper TiOF2 kan transformeren in TiO2 holle nanoboxen assemblage door zes geordende nanosheets met blootgestelde hoogenergetische (001) facetten. De perifere tetracarboxylgroep van ZnTCP vormde de sterke esterbinding gecombineerd met de drager TiO2 -HNB's (Vergelijking 4).
$$ {\displaystyle \begin{array}{l}{\mathrm{TiO}\mathrm{F}}_2+2{\mathrm{CH}}_3{\mathrm{CH}}_2\mathrm{O}\ mathrm{H}\circledR {\mathrm{TiO}}_2+2\mathrm{HF}+2\mathrm{CH}={\mathrm{CH}}_2\left({\mathrm{CH}}_3{\ mathrm{CH}}_2{\mathrm{OCH}}_2{\mathrm{CH}}_3\right)\\ {}\mathrm{alcohol}\;\overset{\mathrm{uitdroging}}{\to}\;\mathrm{alkene}\ \left(\mathrm{ester}\right)+{\mathrm{H}}_2\mathrm{O}\end{array}} $$ (1) $$ \mathrm{Zn} \left(\mathrm{II}\right)\hbox{-} \mathrm{porphyrin}\hbox{-} \mathrm{COOH}+{\mathrm{CH}}_3{\mathrm{CH}}_2\mathrm {O}\mathrm{H}\circledR \mathrm{Zn}\left(\mathrm{II}\right)\hbox{-} \mathrm{porphyrin}\hbox{-} {\mathrm{COOCH}}_2{ \mathrm{CH}}_3+{\mathrm{H}}_2\mathrm{O} $$ (2) $$ {\mathrm{TiO}\mathrm{F}}_2+{\mathrm{H}}_2\mathrm {O}={\mathrm{TiO}}_2\left(\mathrm{anatase}\ \mathrm{crystal}\right)+2\mathrm{HF}\left(\mathrm{in}\ \mathrm{situtransformation} \right) $$ (3) $$ \mathrm{Zn}\left(\mathrm{II}\right)\hbox{-} \mathrm{porphyrin}\hbox{-} \mathrm{CO}\mathrm{OH }+{\mathrm{TiO}}_2\hbox{- } \mathrm{O}\mathrm{H}=\mathrm{Zn}\left(\mathrm{II}\right)\hbox{-} \mathrm{porphyrin}\hbox{-} \mathrm{CO}\hbox {-} \mathrm{O}\hbox{-} {\mathrm{TiO}}_2 $$ (4)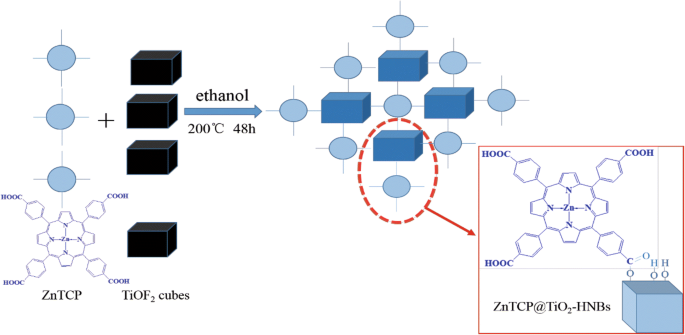
Het voorgestelde vormingsproces van Zn(II)-porfyrine maakte TiO2 . gevoelig -HNB's (ZnTCP@TiO2 -HNB's)
XRD-analyse
Het is algemeen aanvaard dat de kristalvlakken van TiO2 -gebaseerde katalysatoren spelen een zeer belangrijke rol vanwege hun fotokatalytische eigenschappen. Afbeelding 4 toont de XRD-patroonanalyse van alle voorbereide monsters. Kijkend naar de curve ten opzichte van TiOF2 voorloper, {100}, {200} en {210} kristalvlakken van TiOF2 overeenkomend met 2θ =-24,05°, 48,34° en 54,45° werden duidelijk waargenomen. Voor alle TiO2 monsters, een brede piek bij 2θ = 25,37° werd waargenomen, wat overeenkomt met de {101} vlakdiffractie van anatase TiO2 (JCPDS No. 21-1272), anders dan 24,05°, die wordt toegeschreven aan de diffractiepiek van TiOF2 , gaf aan dat alle monsters het topotactische transformatieproces met TiOF2 . met succes hebben voltooid als sjabloon [40]. Vanwege F − ionen als vormcontrolemiddel, er was geen rutiel TiO2 patronen gevormd tijdens het gesynthetiseerde proces. Vergeleken met TiO2 -HNB's (T0p), de diffractiepieken rond {101} en {200} kristalvlak van Zn(II)-porfyrine-gesensibiliseerd TiO2 -HNBs-monsters worden scherper, impliciet hoger in de mate van kristallisatie, wat wordt toegeschreven aan het feit dat de toename van de hoeveelheid Zn(II)-porfyrine leidde tot de concentratietoename van chemische esterbindingen tussen de TiO2 oppervlakte-hydroxylgroep en perifere tetracarboxylgroep van ZnTCP. De andere karakteristieke diffractiepieken van TiO2 monsters in XRD-patronen vertoonden geen verschuivingen of veranderingen in piekvorm, wat erop wees dat ZnTCP-modificatie en sensibilisatie de kristalstructuur van TiO2 niet veranderde -HNB's.
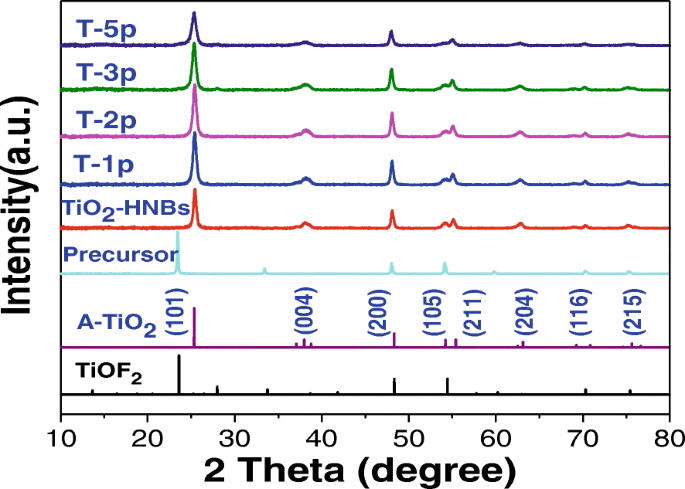
XRD-patronen van voorbereide monsters
Om de kristallietgroottes van ZnTCP@TiO2 . verder te onderzoeken -HNBs-monsters, werd de Scherrer-vergelijking gebruikt op basis van hun diffractiepiek [41, 42]. Zoals beschreven in tabel 1, gaven alle monsters hun gemiddelde deeltjesgrootte aan bij ongeveer 260 nm volgens structurele analyse, in overeenstemming met de SEM- en TEM-metingen. Echter, de “d ” ruimtewaarde van ZnTCP@TiO2 -HNBs-monsters namen niet toe in vergelijking met TiO2 -HNB's, wat de eenheidsceldimensie van de voorbereide ZnTCP@TiO2 impliceert -HNBs-katalysator zou waarschijnlijk niet veranderen tijdens Zn(II)-porfyrine-gesensibiliseerde procedure.
UV-vis-spectroscopie
Afbeelding 5 toont het UV-vis spectrum van de oplossing van ZnTCP in 5 × 10 −4 mol/L DMF-oplossing (a) en de UV-vis diffuse reflectiespectroscopie (DRS) van ZnTCP@TiO2 -HNBs-samples en BaSO4 werd gebruikt als het referentiemonster (b). As Fig. 5a displayed, the strong 432 nm absorption peak was ascribed to the characteristic B-band absorption of porphyrin rings, whereas weaker 561 nm and 600 nm peaks in the visible region were attributed to the characteristic Q-band absorption of ZnTCP, implying the successful synthesis of porphyrin ring. Compared to Fig. 5a, the Q-band absorption peaks of ZnTCP was red-shifted to 660 nm into the longer wavelength visible region after sensitization to TiO2 . Because there is a presented strong interaction force between zinc porphyrins and TiO2 -HNBs after hydrothermal reaction, which provides favorable prerequisites for the degradation of organic dyes under the visible-light irradiation. It can be found that TiO2 -HNBs displayed less absorption above its fundamental absorption edge (> 400 nm), while Zn(II) porphyrin-sensitized TiO2 samples displayed an obvious broad visible-light absorbance, as described in Fig. 5b, and the absorption intensities of ZnTCP@TiO2 -HNBs samples were gradually enhancing much stronger as Zn(II) porphyrin amount increased. In addition, the color of as-prepared samples become deepened gradually from white to blue purple with the increasing of mass ratio of ZnTCP/TiO2 -HNBs, and it was widely accepted that the purple coloration cause Zn(II) porphyrin-sensitized samples resulted into enhanced visible-light absorption. To further investigate the bandgap in each sample, the energy gap (eV) threshold was obtained using the transversal method, and the graph of the transformed Kubelka-Munk function against the photon energy for samples T-2p and TiO2 -HNBs are displayed in Fig. 5c. According to the transformed Kubelka-Munk function plot, the energy gaps of E g (T-2p) en E g (TiO2-HNBs) are 2.83 and 3.08 eV, respectively. The reduced bandgap arises because ZnTCP can form a local energy state between the valence and conduction bands as ZnTCP concentration increases, which reduce the forbidden band width and the electron transition energy. Meanwhile, we find that the ZnTCP self-doped samples also exhibit very strong UV absorption, which indicates ZnTCP self-doping not only enhances the visible-light response of the catalysts, but also improves their UV light absorption. Thus, it can be predicted that more photo-generated electrons and holes can be excited and can participate in photocatalytic reactions, which is attributed to the increased probabilities of activation by UV and visible light.
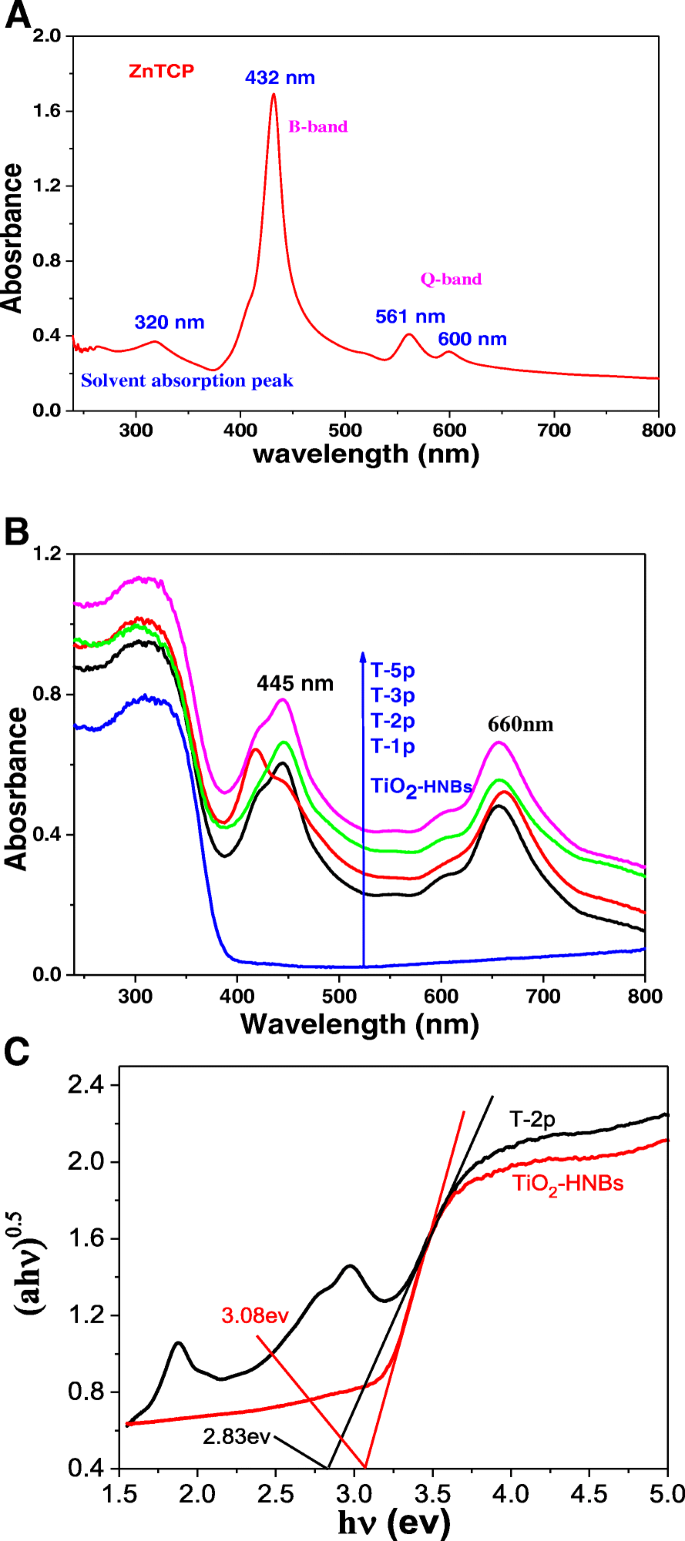
een UV-Vis spectra of ZnTCP in 5×10 −4 mol/L DMF solution. b UV-vis diffuse reflectance spectra (DRS) of all the samples. (c ) Estimated optical absorption edges for the T-2p and TiO2-HNBs
FT-IR Spectra Analysis
FT-IR spectra analysis is a powerful tool to identify characteristic functional groups [43]. Figure 6 displays the FT-IR spectra of ZnTCP, TiO2 -HNBs, and T-2p. Looking at the spectra relative to ZnTCP, the broad band at 3443 cm −1 is assigned to the stretching vibration of the -OH in the peripheral t carboxyl groups, the characteristic absorption peak of C=O at 1720 cm −1 . The stretching vibration of Ar-H bond is clearly observed at 2995 cm −1 . Furthermore, the spectra of ZnTCP show the characteristic absorption peak of benzene ring at 1620 and 1469 cm −1 relative to ZnTCP [44].
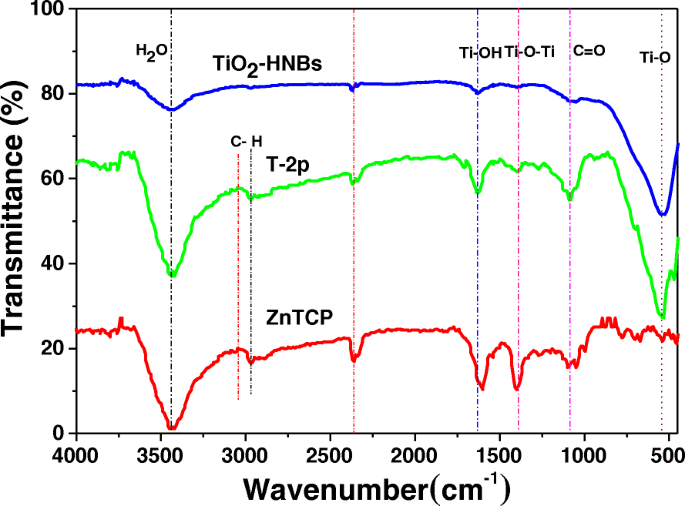
FT-IR spectra of TiO2 -HNBs, ZnTCP, and T-2p sample
Seeing from the spectra relative to TiO2 -HNBs, the stretching vibration and bending vibration of the absorption H2 O and Ti–OH group on the surface of samples were found about 3446 cm −1 and 1627 cm −1 , respectievelijk. The stretching vibration of Ti-O-Ti is observed at 1388 cm −1 , and the adsorption peaks around 521 cm −1 is assigned to the bending vibrations of Ti-O bond.
Compared to ZnTCP and TiO2 -HNBs, the T-2p spectra features the characteristic stretching/bending vibration of the Ar-H bond at 2996 cm −1 and 1386 cm −1 attributed to Ti-O-Ti bond. Furthermore, the spectra of T-2p shows the characteristic absorption peak of phenyl ring at 1621 and 1468 cm − 1 corresponding to ZnTCP, indicating the existence of ZnTCP on the surface of sensitized TiO2 [44]. However, the signal corresponding to -OH vibration peak disappeared, which demonstrates the formation of ester chemical bond between ZnTCP and TiO2 . The stretching vibration of the C=O bond of ester group is clearly observed at 1713 cm −1 [45], further confirmed ZnTCP combined to TiO2 -HNBs composites. The above information indicates that the TiO2 -HNBs surface has been successfully functionalized with ZnTCP molecules. After sensitization, the spectra of T-2p show an increased intensity of the stretching vibration peak at 520 cm −1 and the characteristic absorption bands in the region of 1200~1060 cm −1 attributed to the CO-O-Ti bond resulting from the esterification between COOH of ZnTCP and OH of TiO2 [46]. This reveals that the strong interaction (conjugated chemical bonds) between ZnTCP and TiO2 -HNBs was established rather than simple physical adsorption. The results displayed on FT-IR spectra were in accordance with the photocatalytic performance of these catalysts, suggesting that the strong conjugated chemical bonds between ZnTCP and TiO2 greatly contribute to the improvement of the photocatalytic activity as a transferring electrons bridge.
XPS Analysis
To further investigate chemical environments of as-prepared catalysts, the X-ray photoelectron spectra (XPS) measurements of TiO2 -HNBs and T-2p were carried out [43]. As shown in full spectra of Fig. 7a, two elements including Ti and O were both observed at the corresponding positions, respectively. Figure 7b–f displayed the high-resolution XPS spectra of the corresponding element of two samples.
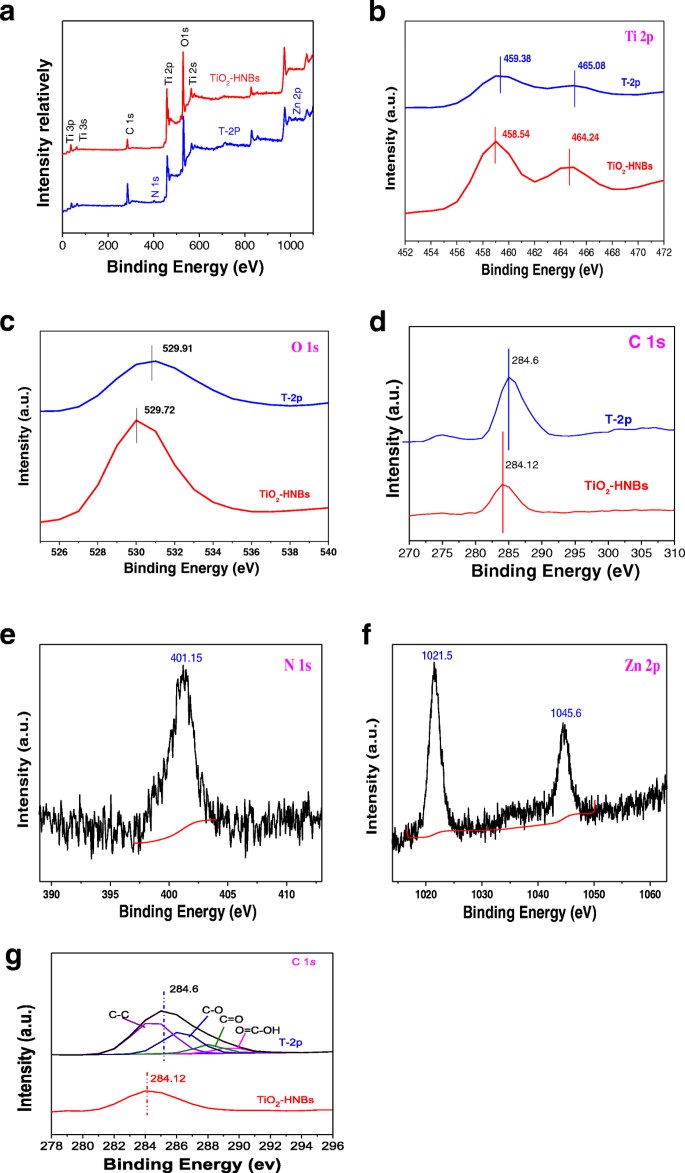
een The full spectra of all kinds of elements in sample TiO2 -HNBs and T-2p; b the high-resolution Ti 2p XPS signals of TiO2 -HNBs and T-2p; c the O1s XPS signals of TiO2 -HNBs and T-2p; d the C1s O1s XPS signals of TiO2 -HNBs and T-2p; e the N1s XPS signals of T-2p; v the Zn 2p XPS signals of T-2p; g the C XPS-peak-differentation-imitating analysis
The high-resolution Ti 2p XPS signals of TiO2 -HNBs in Fig. 7b consisted of two binding energy levels of Ti 2p1/2 and 2p3/2 at 464.24 and 458.54 eV with a separation energy of about 5.70 eV. However, the binding energy levels of Ti 2p1/2 and 2p3/2 XPS peaks of T-2p exhibited at 465.08 and 459.38 eV, which shift 0.84 eV toward the lower energy region compared to TiO2 , indicative of a partial charge transferred from the surface C=O ester moiety to Ti 4+ centers, which was probably due to the strong interactions between ZnTCP molecules and TiO2 .
As shown in Fig. 7c, the O1s XPS signals of TiO2 -HNBs and T-2p displayed at 529.72 and 529.91 eV respectively, with a deviation energy of nearly 0.19 eV. The shifting of lattice oxygen peaks in T-2p toward lower energy, compared to TiO2 standard, further verified the different chemical environment due to ZnTCP sensitization. As displayed in Fig. 7d, the C 1s of T-2p appeared the distinct peaks at 284.6 eV, which were ascribed to C=O (and COO) bonds in the peripheral tetracarboxyl group, further confirmed that ZnTCP was well bonded on the surface of TiO2 . It was found that the weak peak at 401.15 eV of T-2p in Fig. 7e was attributed to N1s of the N=N- bond in the porphyrin ring. The Zn 2p binding energy signals of T-2p appeared at 1021.5 eV and 1044.46 eV in Fig. 7f, which are basically consistent with the complex molecular structure of ZnTCP, further implying it well impregnated onto the surface of TiO2 . Figure 7g shows the XPS spectra of C 1s region for samples. In Fig. 7g, the peak with a binding energy of 284.6 eV can be attributed to the C–C bonds, while the deconvoluted peaks centered at the binding energies of 286.5, 288.3, and 289.6 eV can be assigned to the C–O, C=O, and O=C–OH functional groups, respectively. C 1s spectra for the ZnTCP@TiO2 -HNBs composite are shown in Fig. 7. In the spectrum of ZnTCP@TiO2 -HNBs, all peaks from oxygen-containing functional groups decreased dramatically in intensity or even disappeared entirely, indicating a significant reduction of GO by solvothermal treatment. In addition, an additional shoulder peak was found, which was usually assigned to the formation of a chemical bond between a carbon atom and a titanium atom in the lattice of TiO2 -HNBs, which resulted information of Ti–O–C bonds.
Photocatalytic Activity of ZnTCP@TiO2 -HNBs Catalysts
The photocatalytic activity of ZnTCP@TiO2 -HNBs was evaluated by measuring the degradation with RhB as a probe molecule. Figure 8a exhibits the degradation curve of RhB under simulated sun light conditions with as-prepared ZnTCP@TiO2 -HNBs catalysts. It is clearly observed that, after the adsorption-desorption equilibrium process, the self-degradation of probe molecule RhB can be negligible and the visible-light degradation activities of ZnTCP-sensitized TiO2 -HNBs samples indicates much higher photocatalytic efficiencies than TiO2 -HNBs and ZnTCP under visible-light irradiation (λ ≥ 420 nm), which depends on the concentration of RhB versus visible-light irradiation time. The T-2p sample shows highest photocatalytic activity with RhB degradation rate of 99% after 2 h under visible-light irradiation, resulting from its enhanced visible-light response. However, the TiO2 -HNBs (T-0p) shows little decomposition rate of RhB under the same reaction conditions. In this process, the order of degradation rate is T-2p> T-3p> T-1p> T-5p> ZnTCP>TiO2 -HNBs.
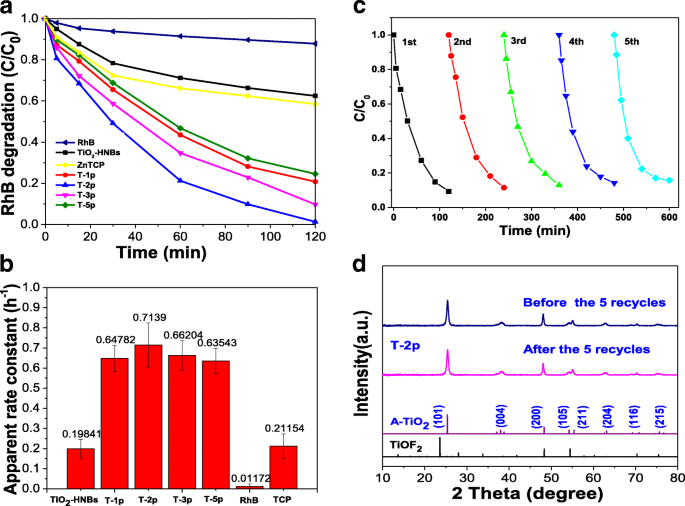
een The C t /C0 vs. time curves of RhB photodegradation under visible-light irradiation. b The apparent first-order rate constant of RhB photodegradation. c Cyclic degradation curve of T-2p. d The XRD before and after recycles of T-2p
It is well accepted that the slopes for the curve of RhB intensity versus illumination time (rate constant) represent the photodegradation activity of the samples. Therefore, the exponential curve of the degradation RhB under visible-light irradiation was fitted in Fig. 8b, and the degradation kinetics of RhB was described by the first-order kinetics curves. Degradation experiments followed the first-order kinetic equation ln(C0 -C) = − Kt + b (in which K is the apparent first-order rate constant and t is the reaction time). By comparing the rate constants (K values) of ZnTCP-sensitized TiO2 -HNBs catalysts, we can see that the rate constants of these catalysts increase first and then decrease with the ZnTCP/TiO2 -HNBs mass ratio increase. Observed from the rate constants histogram (Fig. 8b), we can find that T-2p reveals the highest visible-light photocatalytic activity (rate constant of 0.7139), which is 3.6 times higher than that of TiO2 -HNBs (T-0p) (rate constant of 0.19841); furthermore, the photocatalytic rate constants of other ZnTCP@TiO2 -HNBs samples are basically larger than that of TiO2 -HNBs.
The possible reasons is that may be because ZnTCP-sensitized TiO2 -HNBs can extend the absorption wavelength range of TiO2 -based catalysts, reducing the bandgap of TiO2 , and facilitate the effective separation of the electron-hole pairs generated by TiO2 -HNBs after absorbing visible light, thereby improving the utilization of electron-hole pairs. However, too much ZnTCP-sensitized parts may result in the increase of defects on the surface of TiO2 -based catalyst, resulting in a rapid recombination center of photogenerated electrons and holes, thereby decreasing the photocatalytic activity.
In order to investigate the long-term stability of as-prepared ZnTCP@TiO2 -HNBs, the photocatalysts were recycled after each photocatalytic degradation experiment and then were reused in the next run after washing treatment. Figure 8c shows sample T-2p were recycled to 5 rounds after bleaching RhB under visible-light irradiation; as the number of photodegradation cycles increased, the photocatalytic rate of RhB still maintained a high catalytic activity, indicating that the active site of ZnTCP@TiO2 -HNBs surface did not decrease, and the composite photocatalyst has good photocatalytic stability under visible-light irradiation. The strong bridging ester bond-linking leads to a slower decrease of the degradation efficiency for sample T-2p. These results further demonstrate that ZnTCP can establish a steady chemical bridging bond-linking interaction between ZnTCP and TiO2 -HNBs, thus enhancing the visible-light photocatalytic performance, stability, and recyclability of ZnTCP@TiO2 -HNBs. XRD shows that the T-2p is still anatase type and did not change the crystal type after many cycles, further verifying the stability of the catalyst (Fig. 8d).
PL Analysis
In order to study the active species generated during photocatalytic degradation, the coumarin, 4-chloro-7-nitrobenzo-2-oxa-1,3-diazole (NBD-Cl), and 1,3-diphenylisobenzofuran (DPBF) were used as the hydroxyl radical (•OH), superoxide radical (•O2 − ), and singlet oxygen ( 1 O2 ) fluorescent probe molecules, respectively. Figure 9a displays the active species of hydroxyl radical detection map with T-2p as photocatalyst. As shown in Fig. 9a, the fluorescence emission wavelength was centered at 450 nm and the fluorescence intensity gradually increased, indicating the concentration of •OH group was increasing with the increase of illumination time. The reason is due to the fact that the continuously generated •OH active group and coumarin react to obtain 7-hydroxycoumarin, the amount of the reaction is gradually increased, and the fluorescence intensity is gradually increased [44, 45].
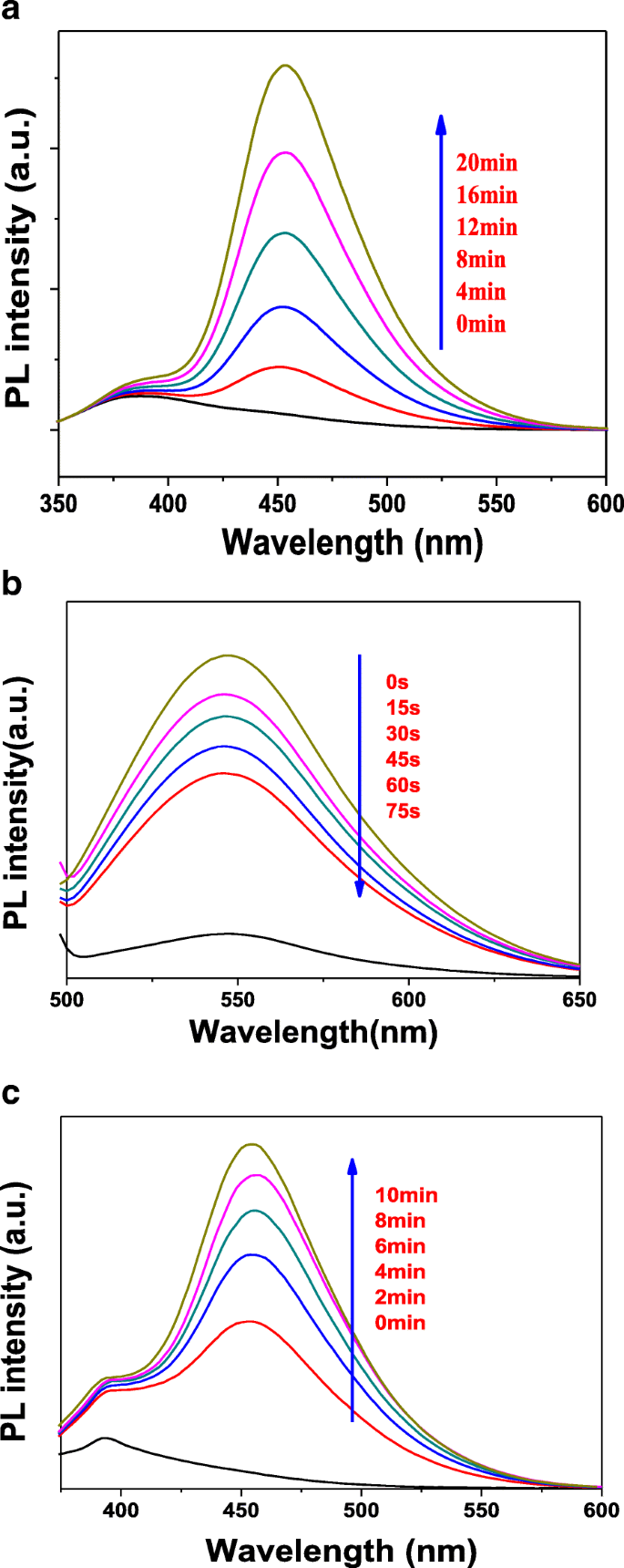
Fluorescent detection of active species •OH (a ), •O2 − (b ), and 1 O2 (c ) with T-2p as photocatalyst
It is well accepted that quantification of superoxide ion concentration can be monitored its reaction with 4-chloro-7-nitrobenzo-2-oxa-1,3-diazole (NBD-Cl) by measuring its fluorescence emission intensity at 550 nm using an excitation wavelength of 470 nm. According to Fig. 9b, it can be found that the fluorescence emission wavelength was centered 550 nm and the fluorescence intensity gradually decreased with the increase of illumination time. In the presence of NBD-Cl, the degradation rate is not obviously increased. The results indicate that •OH radical is hardly generated, while •O2 − is the significant active species generated in the system. More e − can react with O2 to form superoxide radical •O2 − instead of the rapid recombination with h + , which further improves the photodegradation activity and testifies the catalyst can generate the active species •O2 − .
In addition, a typical fluorescence method for detecting singlet oxygen ( 1 O2 ,) by using 1,3-diphenylisobenzofuran (DPBF) as a probe molecule is proposed, which exhibited a strong fluorescence spectrum with a maximum at 455 nm when excited at 410 nm. As shown in Fig. 9c, the fluorescence emission wavelength was centered 455 nm and the fluorescence intensity gradually increased with the increase of illumination time. Based on the above observation, it can be concluded that the photocatalyst produces a certain amount of singlet oxygen in the light condition. To sum up, the ZnTCP@TiO2 -HNBs catalyst can generate •OH, •O2 − , and 1 O2 active species during the photodegradation process under visible-light irradiation.
Electrochemical Analysis
To attain more insights into photocatalytic transformation for oxidation degradation organic pollutants, the electrochemical properties of ZnTCP and ZnTCP@TiO2 -HNBs (T-2p) in DMF were investigated with cyclic voltammetry (CV) on a glassy carbon electrode using tetrabutylammonium perchlorate as supporting electrolyte. It can be clearly seen that two complete oxidation and reductions processes for ZnTCP observed from the potential window are shown with blue dashed lines in Fig. 10. Furthermore, ZnTCP@TiO2 -HNBs (T-2p) distinctly displayed similar two oxidation processes resulted from the ZnTCP center with red dashed lines [39]. The oxidation-reduction electrochemical behavior of ZnTCP complexes mainly occurred on the porphyrins ring and did not involve the change in the valence state of the central metal ions, which were good agreement with our previous reported [47]. However, the oxidation degradation reaction of ZnTCP@TiO2 -HNBs (T-2p) was very similar as that of ZnTCP, which indicated the photocatalytic transformation mainly happened on the porphyrin ring, not relative to the center zinc ion and TiO2 -HNBs. These results further confirm that the strong interaction, such as conjugated ester chemical bonds between TiO2 -HNBs and ZnTCP, was established rather than simple physical adsorption interaction.
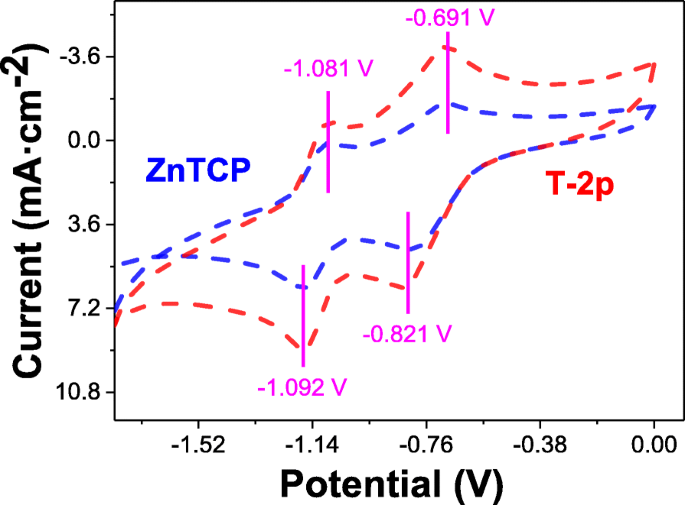
Cyclic voltammetry analysis of 1.0 × 10 −3 mol dm −3 of ZnTCP and T-2p on Pt in 0.1 mol dm −3 TBAP/DMF vs. SCE. Scan rate:0.1 V s −1
Proposed Probable Photocatalytic Mechanism
Much effort has been devoted to illustrate the photocatalytic mechanism for oxidation degradation organic pollutants. In order to speculate the photodegradation mechanism, the main oxidative species in the photocatalytic process was firstly detected through radical and hole trapping experiments by using EDTA (hole h + scavenger), p -benzoquinone (BZQ, superoxide radical •O2 − scavenger), iso -propyl alcohol (IPA, hydroxyl radical •OH scavenger) and 1,3-diphenylisobenzofuran (DPBF, singlet oxygen 1 O2 scavenger). Figure 11 shows the Ct/C0 vs. time curves of different reactive species scavengers on the photodegradation of RhB under visible-light irradiation.
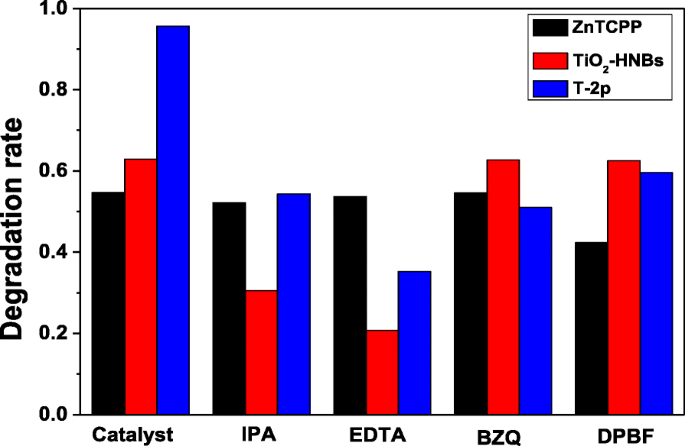
The C t /C0 vs. time curves of different reactive species scavengers on the photodegradation of RhB under visible-light irradiation
As displayed in Fig. 11, the photocatalytic degradation of RhB was apparently restrained after the injection of the active species scavenger. Taking the decreasing photodegradation rate into consideration, it can be found that RhB degradation rate with ZnTCP as a photocatalyst mainly depended on active species of 1 O2 group according to the 1,3-diphenylisobenzofuran (DPBF) detection results. Furthermore, RhB photodegradation rate with TiO2 -HNBs as catalysts mostly depended on active species of h + and •OH group. However, it is very clear that RhB photodegradation rate with ZnTCP@TiO2 -HNBs (T-2p) as catalysts was influenced by all active species of hole (h + ), hydroxyl radical (•OH), superoxide radical (•O2 − ), and singlet oxygen ( 1 O2 ), further confirming ZnTCP@TiO2 -HNBs (T-2p) can generate these active species during the photodegradation process.
The probable photodegradation mechanism of organic dyes with ZnTCP-sensitized TiO2 -HNBs as catalysts under visible-light irradiation is shown in Fig. 12. The degradation process generally consists of three moieties. The first part mainly involves the generation of singlet oxygen ( 1 O2 ). When irradiated by simulated sunlight, the inspired electrons of ZnTCP are transferred from the ground state of porphyrin [Pp] to the excited singlet state 1 [Pp]* [47], and then, relaxation of the singlet excited state generates the triplet excited state 3 [Pp]* through a process of intersystem crossing. Electrons from 1 [Pp]* and 3 [Pp]* excited states are trapped by the adsorbed O2 to form singlet oxygen ( 1 O2 ), which can cause RhB degradation into pieces of small organic molecule or mineralization to CO2 .
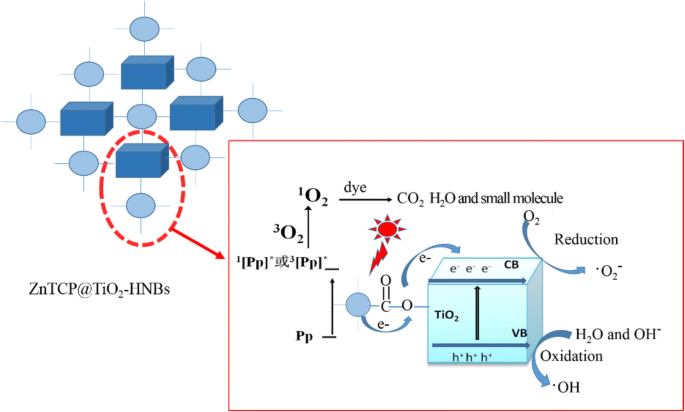
Proposed probable photocatalytic mechanism of ZnTCP@TiO2 -HNBs
In contract, superoxide radical (•O2 − ) can be generated in the second moiety. Biomimetic catalytic moiety of ZnTCP is not only responsible to generate singlet oxygen ( 1 O2 ), but also acts as electron bridges from 1 [Pp]* and 3 [Pp]* excited states transferred to conduction band (CB) of TiO2 -HNBs, which can be trapped further by the adsorbed O2 , resulting in formation of an amount of •O2 − causing photodegradation of RhB present on the surface of TiO2 -HNBs.
On the other hand, in the sensitization of porphyrins, the electrons of TiO2 are excited from valence band (VB) to conduction band (CB) forming electron-hole pairs (e − -h + ) during the visible-light irradiation [48, 49]. The photogenerated holes transfer to the surface of TiO2 reacted with H2 O or OH − , resulting in formation of hydroxyl radical (•OH). The photogenerated electrons are transferred to the surface of TiO2 trapped by the adsorbed oxygen molecule, obtained an amount of superoxide radical (•O2 − ), and these active species (•O2 − , •OH) mainly implement the degradation of organic dyes by its successive formation of intermediaries participated.
Thus, overall, a cooperative mechanism is proposed for the degradation of organic dyes involving three components of photocatalytic system. The enhanced visible-light photodegradation activities of Zn(II)TCP@TiO2 -HNBs compared to ZnTCP and TiO2 -HNBs might be related to synergetic generation of three active species (•O2 − , •OH, and 1 O2 ), resulting in more higher efficient photocatalytic activities.
Conclusions
A facile one-step solvothermal treatment via a topotactic transformation process was employed to synthesize a series of visible-light-driven biomimetic photocatalysts based on ZnTCP-sensitized TiO2 hollow nanoboxes assembled by six ordered nanosheets with high-energy {001} facets dominant exposure. ZnTCP played an important role in formation ester bonds to construct 3D hollow nanoboxes and transfer photogenerated electrons to sensitize TiO2 -HNBs for enhancing visible-light response. Due to synergistic visible photodegradation mechanism of biomimetic catalyst, which can produce not only hydroxyl radical (•OH) and hyperoxygen radical (•O2 − ) from TiO2 , but also singlet oxygen ( 1 O2 ) generated by biomimetic enzyme porphyrin, the photocatalytic degradation RhB rate constants of ZnTCP@TiO2 -HNBs (T-2p) were greatly enhanced with a degradation yield of 99%, much larger (3.6 time) than TiO2 -HNBs under visible-light irradiation. This novel approach is expected to provide a perfect reference for perfect dye-sensitized method to synergistically fabricate other surface modification TiO2 -based composites, which is of great value with promising application for purifying domestic sewage.
Afkortingen
- 3D:
-
Driedimensionaal
- CV:
-
Cyclische voltammetrie
- DRS:
-
UV-vis diffuse reflectance spectroscopy
- IR:
-
Infrared spectra
- PL:
-
Fotoluminescentie
- SEM:
-
Scanning elektronenmicroscoop
- TEM:
-
Transmissie-elektronenmicroscoop
- TiO2 -HNBs:
-
TiO2 hollow nanoboxes
- UV-vis:
-
UV-vis spectroscopy
- XPS:
-
Röntgenfoto-elektronenspectroscopie
- XRD:
-
Röntgendiffractie
- ZnTCP:
-
Zinc(II) meso -tetra(4-carboxyphenyl)porphyrinato
- ZnTCP@TiO2 -HNBs:
-
Zinc(II) meso -tetra(4-carboxyphenyl)porphyrinato-sensitized TiO2 hollow nanoboxes
Nanomaterialen
- Nanovezels en filamenten voor verbeterde medicijnafgifte
- Op weg naar TiO2-nanovloeistoffen:deel 1:voorbereiding en eigenschappen
- Eenvoudige synthese en optische eigenschappen van kleine selenium nanokristallen en nanostaafjes
- One-Pot Green-synthese van met Ag versierde SnO2-microsfeer:een efficiënte en herbruikbare katalysator voor reductie van 4-nitrofenol
- Eenvoudige synthese van gekleurd en geleidend CuSCN-composiet gecoat met CuS-nanodeeltjes
- Eenvoudige synthese van zilveren nanodraden met verschillende beeldverhoudingen en gebruikt als hoogwaardige flexibele transparante elektroden
- Hydrothermische synthese van In2O3 nanodeeltjes hybride tweeling hexagonale schijf ZnO heterostructuren voor verbeterde fotokatalytische activiteiten en stabiliteit
- Gemakkelijke synthese van wormgatachtig mesoporeus tinoxide via verdamping-geïnduceerde zelfassemblage en de verbeterde gasdetectie-eigenschappen
- One-Pot-synthese van Cu2ZnSnSe4-nanoplaten en hun door zichtbaar licht aangedreven fotokatalytische activiteit
- Milieuvriendelijke en gemakkelijke synthese van Co3O4-nanodraden en hun veelbelovende toepassing met grafeen in lithium-ionbatterijen
- Eenstaps sonochemische synthese en fotokatalytische eigenschappen van grafeen/Ag3PO4 Quantum Dots Composites in één stap