Vooruitgang in op ijzeroxide gebaseerde nanostructuren voor toepassingen in energieopslag
Abstract
De vraag naar groene en efficiënte energieopslagapparaten in het dagelijks leven neemt voortdurend toe, wat wordt veroorzaakt door de wereldwijde milieu- en energieproblemen. Lithium-ionbatterijen (LIB's), een belangrijk soort energieopslagapparaten, trekken veel aandacht. Grafiet wordt gebruikt als LIBs-anode, maar de theoretische capaciteit is laag, dus het is noodzakelijk om LIBs-anodes met een hogere capaciteit te ontwikkelen. Toepassingsstrategieën en onderzoeksvooruitgang van nieuwe ijzeroxiden en hun composieten als LIBs-anode in de afgelopen jaren zijn samengevat in deze review. Hierin sommen we verschillende typische synthesemethoden op om een verscheidenheid aan op ijzeroxide gebaseerde nanostructuren te verkrijgen, zoals gasfaseafzetting, co-precipitatie, elektrochemische methode, enz. Voor karakterisering van de op ijzeroxide gebaseerde nanostructuren, met name de in-situ röntgendiffractie en 57 Fe Mössbauer spectroscopie worden uitgewerkt. Verder worden de elektrochemische toepassingen van op ijzeroxide gebaseerde nanostructuren en hun composieten besproken en samengevat.
Grafische samenvatting
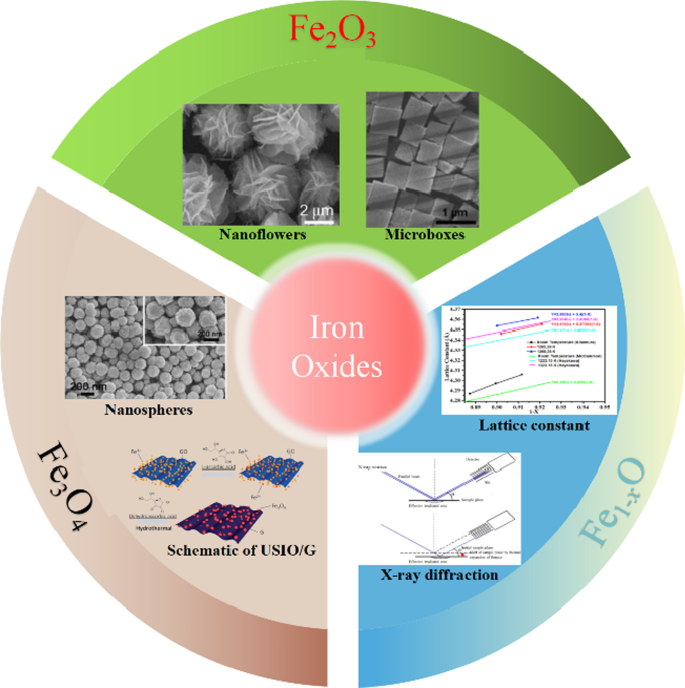
Inleiding
De wereldwijde energie- en milieuproblemen leiden tot een toenemende vraag naar zeer efficiënte groene energie, bijvoorbeeld zonne-energie, brandstofcellen, lithium-ionbatterijen (LIB's) en thermo-elektrische modules, enz. [1,2,3,4,5,6, 7,8,9,10,11]. Onderzoek en ontwikkeling van hoogwaardige en goedkope energieopslagsystemen is een belangrijke oplossing voor deze problemen. Onder de apparaten voor energieopslag met brede toepassingen, zijn LIB's een belangrijke kandidaat voor een zeer effectief energieopslagsysteem [12,13,14,15,16,17,18,19,20,21,22,23,24]. De huidige commerciële grafietanode heeft echter de beperkingen, bijvoorbeeld een relatief lage theoretische capaciteit (372 mA h g −1 ), en er wordt voorgesteld dat sommige elektrochemisch actieve materialen worden toegepast in LIB's [25,26,27,28,29]. In 2000, Poizot et al. [30] meldde dat de overgangsmetaaloxiden (TMO's) worden beschouwd als een soort belangrijke anoden in LIB's vanwege hun hoge theoretische capaciteiten die 2-3 keer groter zijn dan die van grafiet. Daarom trekken TMO's en verwante composieten veel aandacht als LIB's anode. Tijdens het inbrengen/extractieproces van lithium hebben TMO's de volgende reactie [31].
$${\text{M}}_{x} {\text{O}}_{y} + 2j{\text{e}}^{ - } + 2j{\text{Li}}^{ + } \leftrightarrow x{\text{M}}^{0} + y{\text{Li}}_{2} {\text{O}}$$ (1)waarbij M Ni, Cu, Fe, Co, enz. voorstelt. Tijdens het lithium-insertieproces worden deze oxiden gereduceerd door lithium en de composiet bestaande uit metalen clusters gedispergeerd in een matrix van amorf Li2 O wordt gevormd [30, 31].
Onder TMO's zijn op ijzeroxide gebaseerde anodes een soort uitstekende kandidaten met een groot potentieel in LIB's, omdat ze dergelijke voordelen hebben, bijvoorbeeld overvloed, lage kosten en niet-toxiciteit [32, 33]. Echter, net zo vergelijkbaar als andere TMO's, hebben ijzeroxiden die als LIB's-anode dienen, twee kritieke problemen. Een daarvan is de grote onomkeerbare capaciteit, veroorzaakt door ontleding van elektrolyt en vorming van een vaste elektrolytinterface (SEI)-laag in het eerste ontladingsproces. Bovendien is de vorming van Fe en Li2 O is thermodynamisch haalbaar en de extractie van lithiumion (Li + ) van Li2 O is thermodynamisch instabiel [34], aangezien een deel van Li + kan niet worden geëxtraheerd uit Li2 O gevormd in het 1e ontladingsproces. Dit resulteert ook in gedeeltelijk onomkeerbare capaciteit. Een ander probleem is dat hun lage cyclusstabiliteit voornamelijk het gevolg was van een grote volumevariatie en ernstige aggregatie van Fe bij het inbrengen/de-insertie van Li + , wat leidt tot verpulvering van elektroden en snel verval in capaciteit [3]. Om deze problemen op te lossen, wordt er veel moeite gedaan om deze problemen op te lossen en er worden enkele zeer effectieve benaderingen voorgesteld. Voor zover wij weten, is nanostructurering van ijzeroxiden een zeer effectieve strategie [3, 35]. Voor sommige unieke nanostructuren was de variatie in rek en volume het gevolg van insertie/de-insertie van Li + zal grotendeels worden geremd, ook de Li + kan gemakkelijk in elektroden worden gediffundeerd, wat leidt tot aanzienlijk verbeterde elektrochemische prestaties van de anode [11]. Verder worden koolstofhoudende materialen, bijvoorbeeld koolstofvezels (CF's), koolstofnanobuizen (CNT's), grafeen en gepyrolyseerde koolstof, enz. geïntroduceerd voor compositing met ijzeroxiden [36,37,38]. De volumevariatie van composietelektroden in lading/ontlading kan worden gebufferd door deze koolstofhoudende materialen met unieke structuren, waardoor het elektronische contact en de cyclusstabiliteit van ijzeroxidenanostructuren worden verhoogd.
In deze review worden de recent ontwikkelde strategieën en belangrijke onderzoeksupdates over de ijzeroxiden (Fe1−x O, Fe2 O3 , Fe3 O4 ) gebaseerde nanostructuren met toepassingen in LIB's en supercondensatoren worden uitgewerkt en samengevat. We hebben ons in het bijzonder geconcentreerd op de synthese en het ontwerp van de op ijzeroxide gebaseerde nanostructuur, evenals hun elektrochemische prestaties.
Wustite (Fe1−x O)
Wustite (Fe1−x O) is een niet-stoichiometrische verbinding met 0 < x <-0,0464 [11]. Het heeft de kubische structuur van het gesteente en de roosterconstante is ~ 4.330 Å [11]. Fe1−x O, vergeleken met Fe2 O3 en Fe3 O4 , heeft minder toepassingen in energieopslag sinds zijn relatief lage specifieke capaciteit en metastabiele fase onder 843,15 K, die de neiging heeft om te ontleden in Fe en Fe3 O4 . Echter, Fe1−x O, een veelbelovende anode voor LIB's, heeft een hogere elektrische geleidbaarheid dan die van Fe2 O3 en Fe3 O4 .
Synthese en karakterisering
Voor zover we weten, heeft het samenstellen van TMO's met hoogwaardige dekking een groot potentieel voor hoogwaardige LIB's-anode [7]. In LIB's wordt het elektrochemische mechanisme van wustietanode beschreven als de volgende vergelijking [8].
$${\text{FeO}} + 2{\text{Li}}^{ + } + 2{\text{e}}^{ - } \leftrightarrow {\text{Fe}} + {\text{Li }}_{2} {\text{O }}$$ (2)FeO / C-composieten werden gesynthetiseerd met behulp van een gemakkelijke methode door Gao et al. [31]. In hun synthese, α-Fe2 O3 deeltjes met een grootte van 30-120 nm werden gemengd met acetyleenzwart (AB) van verschillende percentages en een uniform mengsel werd verkregen door kogelmalen. Dan, het mengsel van α-Fe2 O3 en AB werd koolstofthermisch gereduceerd bij 800 °C gedurende 10 uur in N2 atmosfeer om uniforme FeO/C-composieten te verkrijgen. De FeO/C-composiet bezit een veel hogere cyclusstabiliteit dan die van Fe2 O3 /AB mengsel. Wanneer het gehalte aan AB 50 gew.% is, is de capaciteit van FeO/C composiet 511 mA h g −1 , hoger dan 396 mA h g −1 van Fe2 O3 /AB. Bovendien is het capaciteitsbehoud na 50 cycli > 96%, duidelijk 70-80% hoger dan dat van Fe2 O3 /AB. Het is aannemelijk dat de superieure elektrochemische prestatie van FeO/C-composiet het gevolg is van zijn hogere elektrische geleidbaarheid, het resultaat van een sterkere verbinding van de FeO- en AB-deeltjes na carbonthermische reductie.
Daarna, in 2016, Jung et al. [11] heeft een kalium (K)-FeO/grafeencomposiet gemaakt als LIBs-anode op basis van K-gedoteerde FeO-nanodeeltjes door thermische diffusie van K in Fe2 O3 /grafeen met behulp van polyolreductie. Rhomboëdrische Fe2 O3 kristallen werden getransformeerd in FeO-kristallen (face-centered cubic, FCC), met een brede d-afstand (5,2 ) van (111) kristalvlakken, door calcinering van K-gedoteerde Fe2 O3 /grafeen. Vergelijking met eerder bestudeerde Fe2 O3 /grafeencomposiet [11], de K-FeO/grafeen vertoonde een ontladingscapaciteit van 1776 mA h g −1 met hoge cyclusstabiliteit gedurende 50 cycli bij een stroomdichtheid van 100 mA g −1 , terwijl Fe2 O3 /grafeen leverde een ontladingscapaciteit van 1569 mA h g −1 . Zelfs bij een hoge stroomdichtheid van 18,56 A g −1 , de capaciteit van K-FeO/grafeen bleef op 851 mA h g −1 na 800 cycli. Dit verschil is veel groter nadat de elektroden langer zijn gecycled bij een hoge stroomdichtheid van 18,56 A g −1 . Zoals getoond in Fig. 1, vergeleken met de Fe2 O3 /grafeen, de K-FeO/grafeenanode heeft een unieke kristalstructuur en een uniek reactiemechanisme. De hoge ontladingscapaciteit van K-FeO/grafeen geeft aan dat specifieke capaciteit door opslag van extra Li + moet worden bijgedragen door de vacatures en brede d-afstand binnen Wustite-roosters door kaliumdiffusie in Fe2 O3 roosters.
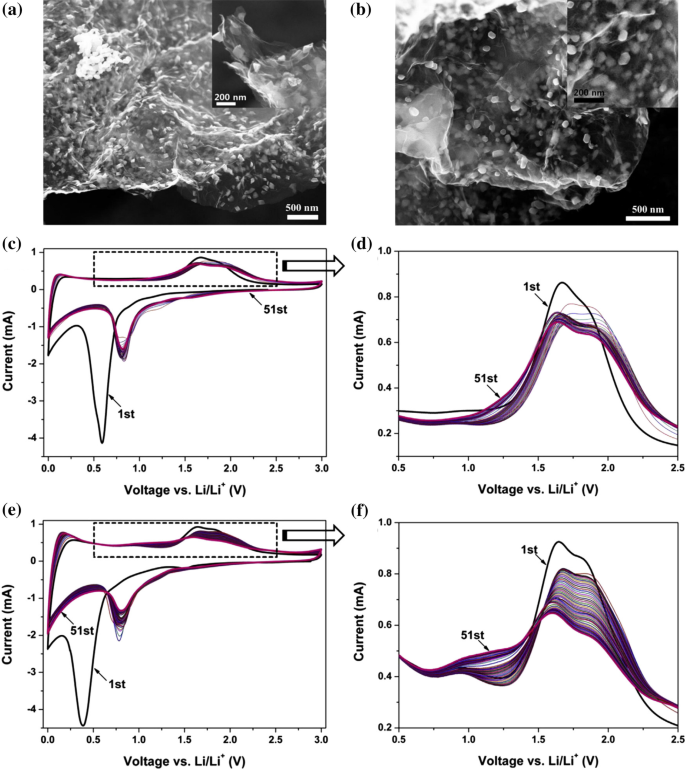
Herdrukt met toestemming van [11]. Copyright, Elsevier B.V.
SEM-afbeeldingen van a Fe2 O3 /grafeen en b K-FeO/grafeen, en de inzet in (a , b ) zijn respectievelijk hun vergrote afbeeldingen. Herhaalde cyclische voltammogrammen van c , d de Fe2 O3 /grafeenelektrode en e , v de K-FeO/grafeenelektrode. Twee elektroden werden continu gescand voor de 51e cycli met een potentiële zwaaisnelheid van 0,5 mV s −1 .
In-situ röntgendiffractie
Door in-situ röntgendiffractie (XRD) kan de informatie over real-time structurele verandering tijdens het reactieproces van het monster en een grote hoeveelheid vergelijkbare informatie in korte tijd worden verkregen. Het kan niet alleen de structurele verandering van het monster tijdens het syntheseproces observeren, het kan ook worden gebruikt om de overeenkomstige structurele verandering van het monster te detecteren bij verschillende temperaturen onder lading / ontlading tot een bepaald potentieel, wat zeer nuttig is voor het bewaken van het eigenlijke reactiemechanisme van anode/kathode in batterij. Daarnaast bestudeerde onze groep de precieze kennis van de roosterconstante van wustite die nodig is voor het onderzoek naar de fysische en chemische eigenschappen ervan bij hoge temperatuur. De Fe1−x O werd gesynthetiseerd en gekarakteriseerd door röntgendiffractometer bij hoge temperatuur (RINT2000-TTR, Rigaku Denki Co., Ltd.) met parallelle bundels (Fig. 2a) voor het meten van de specifieke diffractiepieken en de relatie tussen de samenstelling en roosterconstante van wustite bij hoge temperatuur wordt ook onderzocht. Het syntheseproces van wustite met de α-Fe (95 gew.%) en Fe3 O4 (99 gew.%) poeders als uitgangsmaterialen is een eutectoïde reactie (figuur 2b). Deze reactie kan plaatsvinden tussen 843,15 en 1673,15 K bij bepaalde Pco/Pco2 aangezien de wustite onstabiel is onder 843,15 K, en de reactanten 19% Fe (wt.%) en 81% Fe3 zijn O4 (gew.%). Figuur 2c toont het XRD-patroon van initiële reactanten (magnetiet en ijzer). De experimentconditie wordt beschreven in Tabel 1. Na het zuiveren van helium (He)-gas (25 ml min −1 ) 60 min in de oven van het XRD-systeem, de reactanten werden verwarmd met een constante snelheid van 10 °C min −1 totdat de temperatuur van de monsters 843,15 K bereikte, die zich ook in de He-atmosfeer bevonden met een stroomsnelheid van 25 ml min −1 . Toen was het He-gas uitgeput en CO/CO2 gas met een bepaalde verhouding (bijvoorbeeld 1:1 en 1:2) werd in de oven gespoeld. Na de kalibratie van hoge temperatuur door Au-vlokken te smelten, kon de werkelijke temperatuur van de monsterhouder worden verkregen. Van 843,15 K tot de gewenste temperatuur (1265,28 en 1365,28 K) was de verwarmingssnelheid 2 °C min −1 en de XRD werd gebruikt om het monster te meten voor bevestiging van de wustietfase. Toen het monster bij de gewenste temperatuur was gereserveerd en waarvan het grootste deel de wustietfase was, werden de diffractiehoeken van de kristalvlakken van wustite gemeten gedurende een periode van 240-420 minuten, waarna de temperatuur van het monster met 50 ° C werd verhoogd en op deze temperatuur bleef gedurende 60 minuten. Na deze procedures daalde de temperatuur van het monster tot de eerder gewenste temperatuur en werd de XRD-meting voor diffractiehoeken van kristalvlakken van wustiet opnieuw uitgevoerd in een periode van 180-240 min.
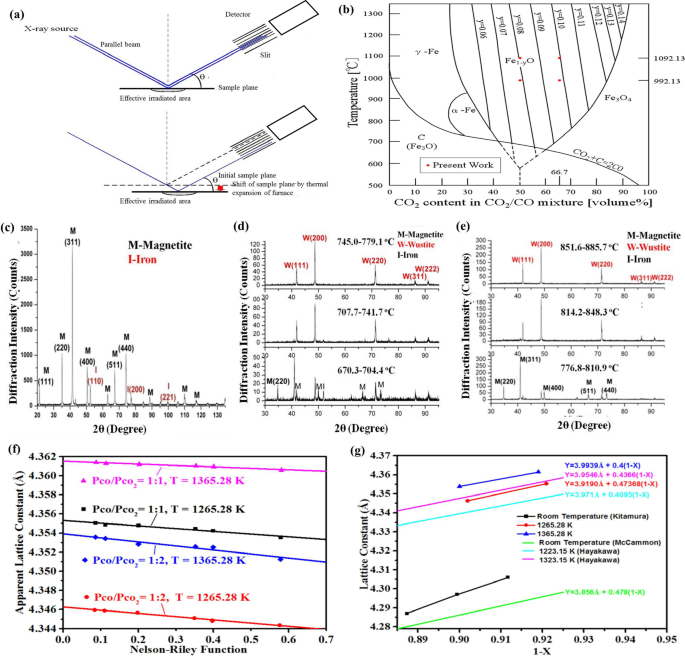
een Schematisch diagram van in-situ XRD. b Evenwicht tussen Fe, Fe1−y O, Fe3 O4 , CO, CO2 , en koolstof. c XRD-patroon van initiële reactanten. d XRD-patroon van het monster gesynthetiseerd op Pco/Pco2 = 1:1. e XRD-patroon van het monster gesynthetiseerd op Pco/Pco2 = 1:2. v De verschillende NR-functie-schijnbare roosterconstanten van wustite gesynthetiseerd bij verschillende temperaturen en Pco/Pco2 . g De resultaten van de roosterconstante van wustite
De roosterconstante kan worden verkregen door een lineaire extrapolatie van de schijnbare roosterconstanten naar nul van deze functie, dat wil zeggen 2 Theta = 180°. De diffractiepieken van (111), (200), (220), (311) en (222) kristalvlakken van wustite zijn geïndexeerd in Fig. 2d, e, en het XRD-patroon van het monster wordt verkregen bij Pco / Pco 2 van respectievelijk 1:1 en 1:2. De relatie tussen schijnbare roosterconstante en Nelson-Riley-functie onder verschillende Pco/Pco2 en temperaturen worden verkregen, zoals weergegeven in figuur 2f. De rechte lijnen vertegenwoordigen de vierkanten die bij de gegevens passen. Door middel van deze rechte lijnen werden de schijnbare roosterconstanten geëxtrapoleerd naar het nulpunt van de Nelson-Riley-functie. Daarom, zoals getoond in Fig. 2f:de resultaten van de echte roosterconstanten verkregen bij verschillende temperaturen en Pco/Pco2 zijn 4.355 Å (1265.28 K, Pco/Pco2 = 1:1), 4,346 Å (1265,28 K, Pco/Pco2 = 1:2), 4,362 Å (1365,28 K, Pco/Pco2 = 1:1) en 4.354 Å (1365.28 K, Pco/Pco2 =-1:2), respectievelijk. Zoals getoond in Fig. 2g, neemt de roosterconstante toe met de toename van x van Fe1−x O, en hoe hoger de temperatuur, hoe groter de roosterconstante. De relatie tussen de samenstelling en de roosterconstante van wustiet bij hoge temperatuur kan worden verkregen als de volgende vergelijkingen. (3) en (4).
$${\text{a}}\,\left( { {\AA} } \right) =3.919 + 0.474\left( {1 - x} \right){ }\left( {1265.28\,{\text { K}}} \right)$$ (3) $${\text{a}}\,\left( { {\AA} } \right) =3.994 + 0.400\left( {1 - x} \right ){ }\links( {1365.28\,{\text{ K}}} \rechts)$$ (4)57 Fe Mössbauer-spectroscopie
De 57 Fe Mössbauer-spectroscopie omvat eigenschappen van de kern, inclusief de energieniveaustructuur van de kern en de chemische omgeving waarin de kern zich bevindt. Daarom kan het dienovereenkomstig worden toegepast om de valentie van atomen, de ioniciteit van chemische bindingen, coördinatiegetal, kristalstructuur, elektronendichtheid en magnetische eigenschappen van het monster te bestuderen. De 57 Fe Mössbauer-spectroscopie wordt veel gebruikt op het gebied van chemie en materialen. Hierin werken we de 57 Fe Mössbauer-spectroscopie voor het karakteriseren van ijzeroxiden. De 57 Fe Mössbauer-spectroscopie wordt gebruikt om verschillende ijzeroxidefasen te onderscheiden en te karakteriseren, en om de lokale omgeving van Fe-atomen in het kristalrooster te volgen [39, 40].
De hyperfijne parameters, zoals isomeerverschuiving (IS), quadrupoolsplitsing (QS), quadrupoolverschuiving (ɛ Q ) en hyperfijn magnetisch veld (B hf ) kan worden verkregen door de positie van de spectraallijnen in het Mössbauer-spectrum te analyseren [41, 42]. De kenmerken van het monster kunnen worden afgeleid uit de breedte en asymmetrie van de spectraallijnen. Door temperatuur- en veldafhankelijkheid van de hyperfijnparameters kunnen ook waardevolle parameters worden afgeleid.
Aldon et al. [42] bestudeerde lithium-geïnduceerde omzettingsreactie van Fe1−x O met 57 Fe Mössbauer-spectroscopie. De hyperfijnparameters (IS en QS) zijn nogal kenmerkend voor FeII-soorten in antiferromagnetische (TN = 198 K [43]) Fe1−x O, met een typische paramagnetische absorptie bij kamertemperatuur (RT). Zoals aangegeven in de 57 Fe Mössbauer spectrum (Fig. 3a), er zijn drie verbrede doubletten met een IS ~ 1 mm s −1 en QS variërend van ~ 0,50 tot 1,50 mm s −1 , en hun relatieve oppervlakten zijn gecorrigeerd voor de magnetische bijdrage van α-Fe. De absorptie-intensiteiten zijn 42, 26 en 15%. Een vierde doublet gecentreerd op IS ~ 0.55 mm s −1 , QS ~ 0,90 mm s −1 met een relatieve oppervlakte van 11% is gebruikelijk voor FeII I soort, zoals verwacht in niet-stoichiometrische Fe1−x O. Van de FeII/FeII I verhouding wordt het aantal vacatures geschat op ~ 0.057 ± 0.008, gesloten tot 0.050 door middel van XRD-karakterisering. Ten slotte bevindt het doublet zich op QS ~ 1,68 mm s −1 en IS ~ 0 mm s −1 , overeenkomend met α-Fe, levert een bijdrage van ~ 6%.
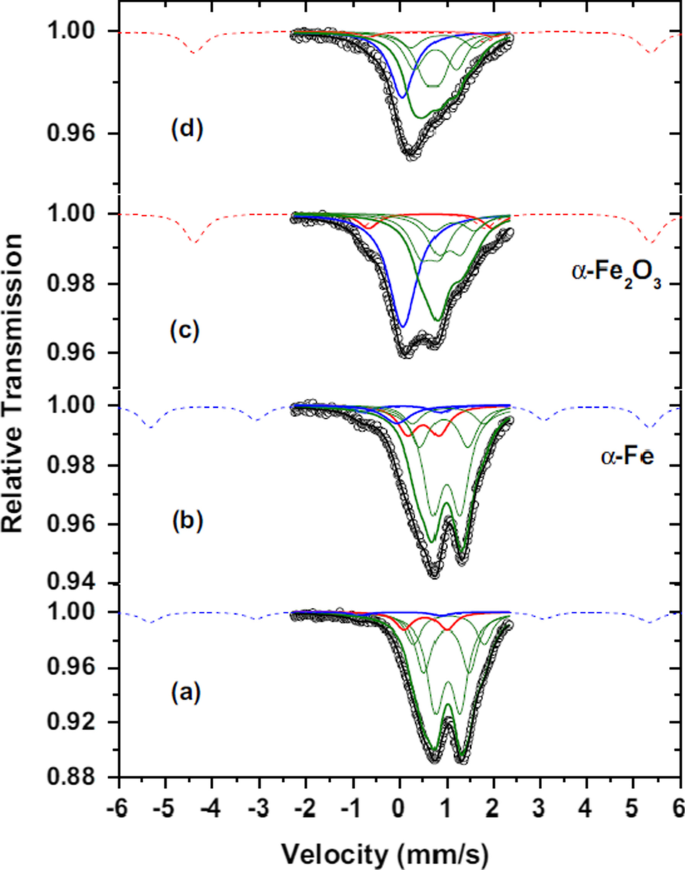
Herdrukt met toestemming van [42]. Copyright, Elsevier Masson SAS
Vergelijking van 57 Fe Mössbauer-spectra bij 300 K van de ongerepte Fe1−x O materiaal (a ), na een opname van 1 Li (b ) en het einde voor ontlading bij 2,16 Li (c ). Groene bijdragen worden toegerekend aan FeII. De dikkere groene lijn is de som van de dunnere die overeenkomt met niet-gereageerd Fe1−x O. Gestippelde blauwe lijn komt overeen met de verwachte toenemende bijdrage van α-Fe van (a ) tot (c ). In het geval van (c ), is het magnetische sextet enigszins verschoven als een gids van het oog. Spectrum (d ) komt overeen met het einde van de eerste lading bij 0,94 Li zonder α-Fe-bijdrage, maar het blauwe singlet met metalen ε-Fe 0 in nanoformaat is nog steeds aanwezig.
Vergelijken met Fe2 O3 en Fe3 O4 , de specifieke capaciteit van Fe1−x O is het laagst. Bovendien is de synthesemethode ingewikkelder. In de meeste gevallen is de reductiereactie bij hoge temperatuur onvermijdelijk [3,4,5,6, 9, 11]. Als gevolg hiervan wordt Fe1−x O is geen ideale LIBs-anode in vergelijking met Fe2 O3 of Fe3 O4 .
Fe2 O3 Gebaseerde nanostructuren
Onder deze ijzeroxiden, vooral Fe2 O3 , trekt de aandacht van veel onderzoekers vanwege de hoge theoretische capaciteit, die kan oplopen tot 1000 mA h g −1 [44]. Bovendien, Fe2 O3 heeft onderscheidende voordelen, zoals hoge weerstand tegen corrosie, lage productiekosten, milieuvriendelijkheid, niet-ontvlambaarheid, niet-toxiciteit en hoge natuurlijke beschikbaarheid [45]. Vanwege deze uitstekende eigenschappen is Fe2 O3 is veelbelovend voor toepassingen in LIB's anode [46,47,48,49,50]. Hierin een beknopt overzicht van recente ontwikkelingen over de synthese, karakterisering en elektrochemische prestatie van Fe2 O3 gebaseerde nanostructuren wordt geboden.
Synthese en karakterisering
In het afgelopen decennium zijn er enorme inspanningen geleverd voor het verkennen van synthetische methoden van Fe2 O3 gebaseerde nanostructuren. In deze sectie hebben we de synthetische methoden van Fe2 . uitgewerkt en samengevat O3 gebaseerde nanostructuren, waaronder gasfaseafzetting [51], op oplossing gebaseerde methode [52], elektrochemische methode [53], thermische behandeling [54] en andere methoden [55, 56]. We hebben ook verschillende synthesemethoden vergeleken.
Bovendien is de 57 Fe Mössbauer spectroscopie karakterisering van Fe2 O3 nanostructuren wordt in detail beschreven. Omdat alleen bepaalde kernen resonantieabsorptie hebben, is de 57 Fe Mössbauer-spectroscopie wordt niet gestoord door andere elementen. Het bereik van de 57 Fe Mössbauer-spectroscopie beïnvloed door een extranucleaire omgeving is over het algemeen binnen enkele nanometers, dus het is zeer geschikt voor het karakteriseren van nanostructuren.
Gasfaseafzetting
Depositie in de gasfase wordt veel toegepast bij de synthese van veel dunne films en andere nanostructuren, zoals Fe2 O3 en andere op ijzeroxide gebaseerde nanostructuren. Chemische dampafzetting (CVD), atoomlaagafzetting (ALD), fysieke dampafzetting (PVD), elektrolytische afzetting en reactief sputteren zijn typische methoden voor gasfaseafzetting [57,58,59,60].
ALD is een unieke manier om dunne films met een hoge kristalliniteit te synthetiseren, en een goedkopere route dan afzetting in de vloeibare fase. In het ALD-proces gaat de chemische reactie van elke laag direct gepaard met de vorige laag. Op deze manier wordt er slechts één laag per reactiecyclus afgezet. Bijvoorbeeld Lin et al. [51] gebruikte ALD om een hoogwaardige ultradunne α-Fe2 . af te zetten O3 film op TiSi2 nanonetten. De 3D zelfgeorganiseerde nanoporeuze dunne films werden vervaardigd door Yang et al. [61] via CVD en geïntegreerd in een heterogene Fe2 O3 /Fe3 C-grafeen. Als LIBs-anode kunnen de snelheidscapaciteit en recycleerbaarheid aanzienlijk worden verbeterd door deze dunne film af te zetten. Cesar et al. [62] afgezette dunne films van met silicium gedoteerd Fe2 O3 dendritische nanostructuren door atmosferische druk CVD (APCVD), die Fe2 . produceerde O3 fotoanodes die water onder zichtbaar licht oxideren met ongekende efficiëntie. De dendritische α-Fe2 O3 nanostructuren vertoonden een macroscopisch oppervlak van 0,5 cm 2 [62]. De verticaal uitgelijnde α-Fe2 O3 nanorods-array wordt gekweekt op een siliciumsubstraat via metaal-organische CVD (MOCVD) door Wu et al. [63]. Wat meer is, Jia et al. [64] gebruikte een radiofrequentie sputterdepositie om α-Fe2 . te fabriceren O3 ultradunne films.
Hoewel de depositie in de gasfase in staat is om Fe2 . van hoge kwaliteit te bereiden O3 op nanostructuren gebaseerde, heeft het ook nadelen. APCVD en MOCVD hebben bijvoorbeeld een hoge toxiciteit en ontvlambaarheid in het proces van voorlopers.
Op oplossing gebaseerde synthetische methode
De op oplossing gebaseerde synthetische methode is gebruikelijk en gemakkelijk om Fe2 . te fabriceren O3 en andere ijzeroxiden. Fe2 O3 met verschillende morfologieën, zoals nanoflowers [65], nanospheres [66], nanoparticles [67], nanorods [68], nanotubes [14], nanorings [69], nanobelts [70], nanoflakes [71], nanowires [72] , nanovezels [73] en microboxen [54], werden gesynthetiseerd door op oplossingen gebaseerde methoden, bijvoorbeeld hydrothermische, solvothermische en sol-gel-benaderingen. Deze methoden zijn zeer eenvoudig en beschikbaar. Zhong et al. [65] gebruikte een solvothermische methode om Fe2 . te fabriceren O3 nanoflowers (Fig. 4) via een door ethyleenglycol gemedieerd zelfassemblageproces. Vayssières et al. [52] rapporteerde de groei van poreus Fe2 O3 nanostaafjes-array op met fluor gedoteerd tinoxide (FTO) geleidend glas door een hydrothermisch proces. Door hydrothermische groei van α-Fe2 O3 voorloper op SnO2 nanodraadstengels, een nieuwe zesvoudige symmetrie vertakte α-Fe2 O3 /SnO2 heterostructuur (Fig. 5) werd gesynthetiseerd [74]. Er is een andere gemakkelijke en economische techniek, de sol-gel-methode, voor het synthetiseren van Fe2 O3 nanostructuren. Woe et al. [68] gebruikte een sol–gel-methode om α-Fe2 . te verkrijgen O3 nanostaafjes door reactie van alomtegenwoordig Fe 3+ in omgekeerde micellen. De nanostaafjes die door dit mechanisme worden verkregen, hebben een lage dimensionaliteit en een groot oppervlak, dat kan worden uitgebreid tot magnetiet en wustiet.
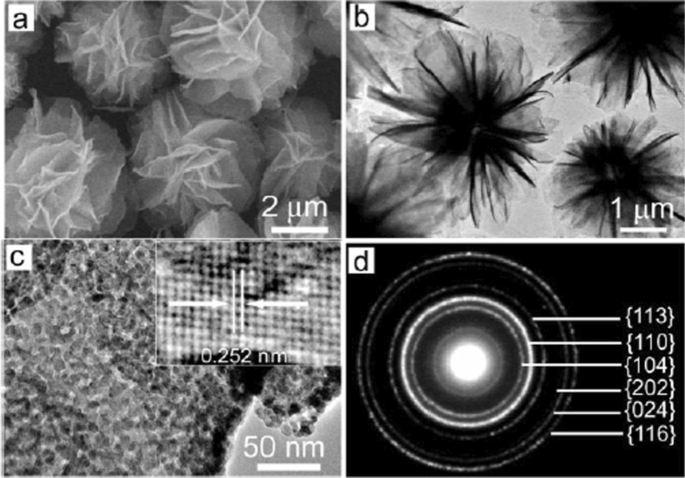
Herdrukt met toestemming van [65]. Auteursrecht, Wiley-VCH
een SEM en b TEM-beelden van de als verkregen α-Fe2 O3 . c TEM-afbeelding met hoge vergroting van het bloemblad van de bloemachtige structuur van de als verkregen α-Fe2 O3 . d SAED-patroon van de verkregen α-Fe2 O3 .
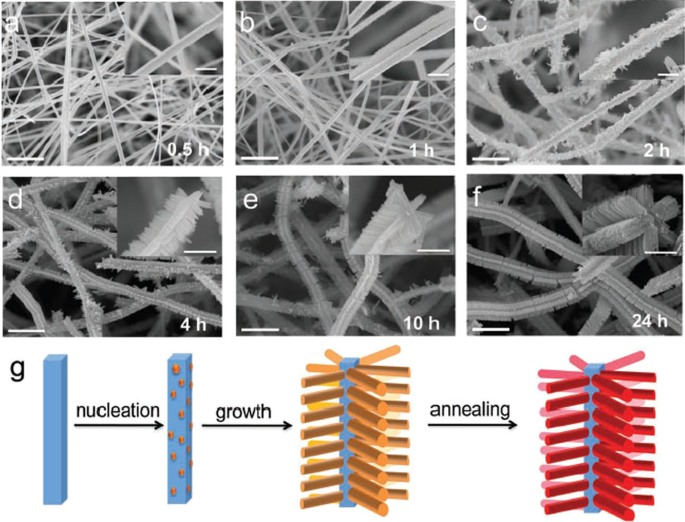
Herdrukt met toestemming van [74]. Auteursrecht, Wiley-VCH
een –f SEM-beelden van de producten (na gloeien) in verschillende reactiestadia door de reactietijd in te stellen. De inzetstukken zijn de bijbehorende vergrote SEM-afbeeldingen. De schaalbalken in de figuren en inzetstukken zijn respectievelijk 2 m en 500 nm. g Schema van het vormingsproces van de hiërarchisch samengestelde α-Fe2 O3 /SnO2 nanocomposiet.
Elektrochemische methode
Elektrochemische methode wordt gebruikt om Fe2 . te synthetiseren O3 nanostructuren, bijv. de elektrochemische afzetting wordt toegepast bij het vervaardigen van Fe2 O3 nanodeeltjes [53]. Door anodisatie van ijzerfolie in ethyleenglycol-elektrolytoplossing met gedeïoniseerd (DI) water en NH4 F bij een spanning van 30-60 V, α-Fe2 O3 nanobuisjes-array werd verkregen [75]. Bovendien wordt elektrochemische anodisatie gebruikt om Fe2 . te synthetiseren O3 nanobuisjes-array [76]. Mao et al. [77] rapporteerde de synthese van Fe2 O3 array met behulp van elektrochemische depositie. In hun onderzoekswerk werd ijzer door elektrochemische afzetting in AAO-sjabloonkanalen afgezet, vervolgens werd het AAO-sjabloon verwijderd door NaOH-oplossing en uiteindelijk werd de ijzeren nanostaafjes-array omgezet in Fe2 O3 reeks. Het kenmerk van dit onderzoek is dat, door de depositieduur te veranderen, de lengte van Fe2 O3 nanostaafjes kunnen nauwkeurig worden gecontroleerd.
Thermische behandeling
De thermische behandeling voor het synthetiseren van Fe2 O3 omvat twee belangrijke benaderingen, thermische oxidatie en thermische pyrolyse. Zhang et al. [54] bereid Fe2 O3 microboxen (Fig. 6) via thermisch geïnduceerde oxidatieve ontleding van pruisisch blauwe (PB) microkubussen bij 350-650 ° C. De solid-state benadering zal een gemakkelijkere manier bieden voor grootschalige synthese van uniforme anisotrope holle structuren in vergelijking met de veelgebruikte oplossingsgebaseerde methode. Fe2 O3 met verschillende morfologieën werden bereid via commanderende thermische oxidatieparameters. α-Fe2 O3 nanostructuren door gemakkelijke thermische behandeling van voorlopers op ijzerbasis worden voorgesteld. Rao en Zheng [71] gebruikten bijvoorbeeld warmtebehandeling om dicht uitgelijnde α-Fe2 O3 nanoflakes-array.
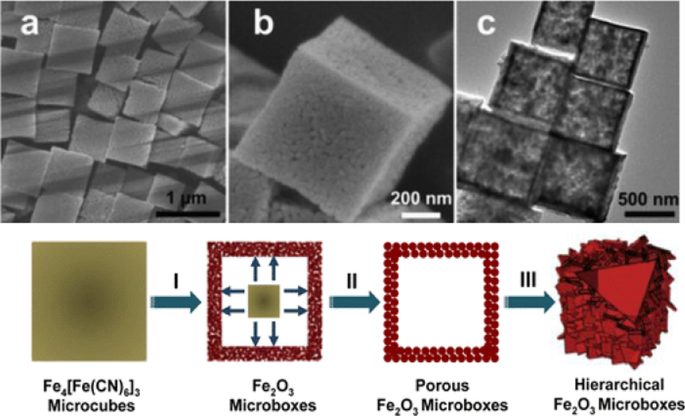
Herdrukt met toestemming van [54]. Copyright, American Chemical Society
een , b FESEM en c TEM-afbeeldingen van holle Fe2 O3 microboxen verkregen bij 350 °C. d Schematische weergave van de vorming van holle Fe2 O3 microboxen en de evolutie van de schaalstructuur met de toenemende calcineringstemperatuur.
Thermische pyrolyse is een andere veelgebruikte methode om Fe2 . af te zetten O3 dunne films. Duret et al. [78] paste deze methode toe om mesoscopische α-Fe2 . te verkrijgen O3 folderfilms door middel van ultrasone spraypyrolyse, terwijl de vervaardigde films een hogere fotoactiviteit hebben dan die vervaardigd door conventionele spraypyrolysetechnieken. Ook de synthese van α-Fe2 O3 nanoflakes, nanoflowers, nanowires en nanorods-array via dampfase-afzetting, vloeistoffase-afzetting en thermische behandeling wordt gerapporteerd [63, 71, 72, 79].
Andere methoden
In 2016 hebben Guivar et al. [55] samengestelde leegstand bestelde maghemiet (γ-Fe2 O3 ) nanodeeltjes gefunctionaliseerd met nanohydroxyapatiet (nanoHAp), met behulp van een typische co-precipitatie chemische route. Opmerkelijk is dat de γ-Fe2 O3 gefunctionaliseerd met nanoHAp gelabeld als γ-Fe2 O3 @HAp wordt gevormd zonder enig thermisch proces als calcinering.
Er zijn veel manieren om Fe2 . te synthetiseren O3 , maar de meeste zijn niet milieuvriendelijk. In 2019 hebben Bashir et al. [56] ontwikkelde een milieuvriendelijke methode om α-Fe2 . te verkrijgen O3 nanodeeltjes, met behulp van Persea Americana zaden extract. Ze gebruikten twee verschillende voorlopers om twee monsters van α-Fe2 . te bereiden O3 , één monster (A) bereid uit Fe(NO3 )3 ·9H2 O, en een ander monster (B) bereid FeCl3 ·9H2 O.
De 57 Fe Mössbauer-spectra van monsters A en B opgenomen bij 300 K (kamertemperatuur (RT)) worden weergegeven in Fig. 7. De 57 Fe Mössbauer-spectroscopie is een zeer waardevolle techniek om het lokale magnetische gedrag en de oxidatietoestand van ijzeratomen in een bepaalde matrix te onderzoeken [80]. Beide monsters onthulden magnetische ordening en vertoonden slechts een enkel sextet dat de magnetisch geordende toestand aangaf. Tabel 2 toont 57 Fe geëxtraheerd Mössbauer parameters bij RT, die IS, ɛ . vermeldt Q en B hf . Beide voorbeelden' B hf boven 51 T zijn gerelateerd aan α-Fe2 O3 [81]. Furthermore, the values of ɛ Q is also consistent with α-Fe2 O3 . Both quadrupole interactions indicate Fe as Fe 3+ since the observed IS of 0.3653 mm/s and 0.3754 mm/s for the samples A and B, respectively, are typical for Fe 3+ [82]. Therefore, the negative values of quadrupole splitting indicate the weak ferromagnetic property of the samples A and B, the characteristic of pure α-Fe2 O3 phase.
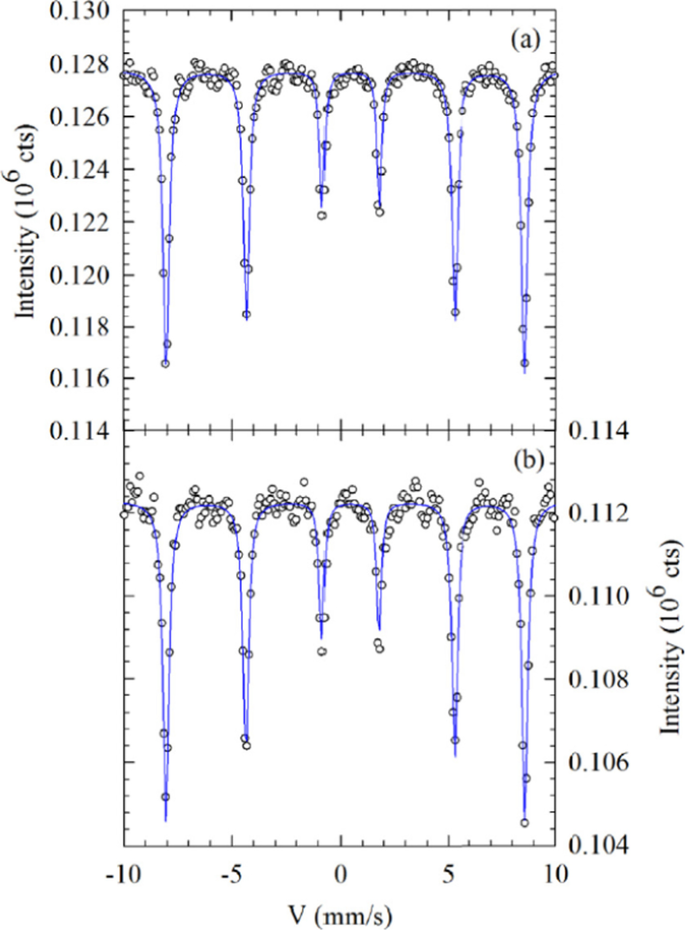
Reprinted with Permission from [56]. Copyright, American Chemical Society
57 Fe Mössbauer spectra recorded at room temperature for α-Fe2 O3 nanodeeltjes. een sample A (ferric nitrate as a precursor) and b sample B (ferric chloride as a precursor).
Electrochemical Performance
The charge/discharge cycling at the voltage window of 0.005–3.0 V (vs. Li + ) under a current density of 200 mA g −1 at RT is shown in Fig. 8a. During the initial discharge process, there is an obvious voltage platform of ~ 0.75 V, and it gradually moved to a voltage plateau of ~ 1.0 V, and remain stable in the second and fifth cycles. Meanwhile, an ambiguous plateau was observed at ~ 1.8 V in the charge process. The first discharge profile qualitatively resembles the results by Larcher et al. [83] and Morales et al. [84] on nanoparticle Fe2 O3 , and Wang et al. [14] on Fe2 O3 nanotubes. The cycling performance of three samples (hierarchical Fe2 O3 microboxes, Fe2 O3 microboxes and porous Fe2 O3 microboxes) is discribed in Fig. 8b. After 30 cycles, hierarchical Fe2 O3 microboxes exhibit the highest reversible capacity of 945 mA h g −1 , follow by 872 mA h g −1 for porous Fe2 O3 microboxes, and finally 802 mA h g −1 for Fe2 O3 microboxes. The results demonstrated that three samples display excellent cycling stability, and the morphology of nanostructured Fe2 O3 plays a significant role in determining the discharge characteristics.
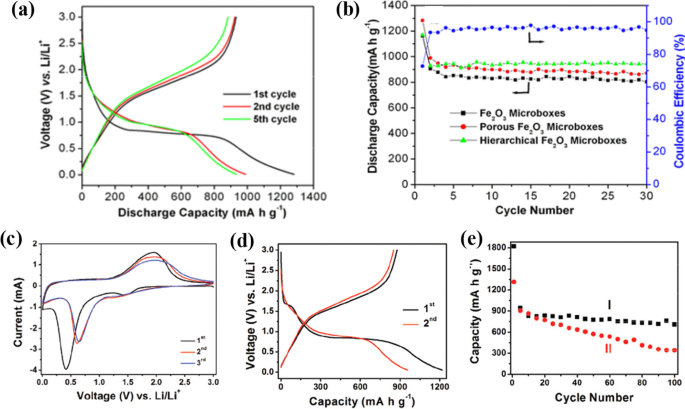
c –e Reprinted with Permission from [85]. Copyright, American Chemical Society
een Discharge − charge voltage profiles of porous Fe2 O3 microboxes obtained at 550 °C. b Cycling performance of Fe2 O3 microboxes (350 °C), porous Fe2 O3 microboxes (550 °C), and hierarchical Fe2 O3 microboxes (650 °C) and Coulombic efficiency of porous Fe2 O3 microboxes (550 °C) over the voltage range 0.01 − 3.0 V vs. Li/Li + at the same current density of 200 mA g -1 . (a , b Reprinted with Permission from [54]. Copyright, American Chemical Society). Electrochemical measurements of the sample. c CVs between 5 mV and 3 V at a scan rate of 0.5 mV s −1 . d Charge–discharge voltage profiles. e Comparative cycling performance of (I) the as-prepared α-Fe2 O3 hollow spheres and (II) α-Fe2 O3 microparticles. All the galvanostatic tests are performed at a constant current rate of 200 mA g −1 between 0.05 and 3 V.
Cyclic voltammogram (CV) is used to characterize the cells with α-Fe2 O3 nanoflakes anode in the 0.005–3.0 V under a slow scan rate (at RT). Li metal is used as the counter and reference electrodes [20], consistent with previously reported results [85]. CV curves of α-Fe2 O3 hollow spheres between 5 mV and 3 V at a scan rate of 5 mV s −1 are presented in Fig. 8c. There are apparent redox current peaks, and demonstrate good reversibility of electrochemical reaction. As shown in Fig. 8d, a distinct voltage plateau can be discovered at ~ 0.75 V, consistent with CV curves. The charge–discharge voltage profiles reflect the lithium storage capacity of α-Fe2 O3 . The first cyclic discharge capacity and the charge capacity is 1219 mA h g −1 and 877 mA h g −1 respectively, which lead to a relatively low irreversible capacity loss of 28%. In the second cycle, the Coulombic efficiency increased quickly to 89%. Cycling performance of two samples are demonstrated in Fig. 8e. The sample (I) exhibits excellent cyclic capacity retention from the second cycle onward. After 100 cycles of charge/discharge, the reversible capacity is still as high as 710 mA h g −1 . Compared with α-Fe2 O3 microparticles, the unique hierarchical α-Fe2 O3 hollow spheres apparently have enhanced Li storage performance, and a more stable cycling capacity retention and a higher reversible capacity are realized. This superior performance can be attributed to the thin nanosheet subunits that provide rapid and efficient transport of Li + , as well as the unique hollow interior that allows the material to effectively buffer the stress generated during charge/discharge process.
Iron oxides are cheap, abundant and environmentally compatible, and Fe2 O3 has excellent electrochemical performance. Some of the above studies have shown that Fe2 O3 based nanostructures can be an alternative anode to replace the presently used graphite in LIBs. The nanostructured Fe2 O3 has great potential in LIBs anode [86,87,88,89,90,91,92,93,94,95]. Recently, several studies about Fe2 O3 anode in asymmetric supercapacitors are reported [96,97,98,99,100]. However, low surface area and poor electrical conductivity are still two critical issues limiting the specific capacitance and power density of Fe2 O3 . For solutions of these problems, CNTs and CNFs are regarded as conductive matrices to load Fe2 O3 nanoparticles for realizing improved performance [101]. Table 3 summarizes some typical Fe2 O3 based nanostructures with their synthesis and electrochemical performance.
Fe3 O4 Based Nanostructures
Magnetite (Fe3 O4 ) and Fe3 O4 based nanostructure composites with pseudocapacitance, high theoretical capacity, environmental friendliness and low cost, are extensively applied to electromagnetic wave absorption [106], LIBs [3, 12, 15], biotechnological devices [107, 108], and supercapacitor [4, 109]. Fe3 O4 nanoparticles is one of the high-performance anodes in electrochemical devices. Unfortunately, Fe3 O4 nanostructures still face some problems, such as a severe volume variation (~ 200%) during the insertion and extraction of Li + and relatively low electrical conductivity, posing negative influence on the cycling stability [19, 110, 111]. From research results, we found that the structure and morphology of Fe3 O4 have a high influence on the electrochemical performance of Fe3 O4 and Fe3 O4 based composites.
Synthesis and Characterization
Recently, nanostructure engineering is demonstrated as a highly-effective approach to obtain improved electrochemical performance of Fe3 O4 and Fe3 O4 based composites. Therefore, various nanostructures including 0D nanoparticles [112], 1D nanorods/wires [113, 114], 2D nanoflakes/sheets [115, 116], 3D hierarchical/porous architectures [117, 118], and hybrid nanostructures of iron oxides [16] are proposed. The electrochemical performance of Fe3 O4 nanostructures can be optimized by rational design of their morphology, composition, porosity and surface characteristics.
Solution phase synthetic method is a facile and rapid way to obtain Fe3 O4 based nanostructures, because of the associative advantages, such as low synthesis temperature (always below 250 °C), easy control of morphology via adjusting hydrothermal conditions (e.g., PH, density of reactant and dosage of active agent, etc.). Solution phase synthetic method includes solvothermal synthesis [119], thermolysis [120], co-precipitation [121], sol–gel process [122, 123], micro-emulsion [124], etc. Simultaneously we compare the pros and cons of these methods.
Solvothermal Synthesis
Solvothermal synthesis, which reacts in a special closed reaction vessel, is one commonly used methods for synthesizing Fe3 O4 . In a hermetic environment, it is a facile method using aqueous solution as reaction medium at high temperature and high-pressure hermetic environment. Fe3 O4 nanostructures with various morphologies (0D, 1D, 2D and 3D) were synthesized applying this approach.
An et al. [119] obtained the Fe3 O4 /graphene nanowires by solvothermal synthesis and calcination with FeCl3 , NH4 VO3 and graphene as precursors. Phase transition of Fe3 O4 /VOx (FVO) after annealing was confirmed by XRD. For the XRD pattern of sample without annealing, all diffraction peaks of FVO and graphene decorated FVO correspond to FeVO4 ·1.1H2 O. For the XRD pattern of sample after annealing, there are no peaks of any vanadium oxides. And the inductive coupled high frequency plasma (ICP) result indicated that the molarity ratio of Fe and V is ~ 0.94:1, confirming the existence of amorphous vanadium oxide.
Mu et al. [125] reported dispersed Fe3 O4 nanosheets on carbon nanofiber by combing the electrospinning and solvothermal process. In this work, Fe3 O4 nanosheets are uniformly attached on the surface of carbon nanofiber with the diameter of about 500 nm.
Fe3 O4 nanoparticle with high specific surface area via FeCl3 and organic solvent ethanolamine (ETA) as precursors is reported by Wang et al. [126]. In this preparation, ETA is critical factor for compounding Fe3 O4 nanoparticles with high specific surface area, and Fe 3+ is gradually reduced to Fe 2+ by ETA during dissolution process, demonstrating that Fe 2+ increased as the increase of ultrasonication time. The ratio of ETA and FeCl3 has a large impact on the nanoscale grain size and specific surface area of Fe3 O4 . And the results showed that the grain size of 20–40 nm is achieved with 60 mL ETA and 6 mmol FeCl3 . When the amount of ETA is 80 mL, smaller nanoparticles (5–10 nm) are obtained.
Another representative work is reported by Chen et al. [127], in which graphene nanosheets decorated with Fe3 O4 nanoparticles (USIO/G) were synthesized using a facile solvothermal process. For the synthesis of USIO composite decorated with reduced graphene oxide (RGO), is used FeCl3 ·H2 O as precursor, then NaHCO3 and L-ascorbic acid were added to form USIO/G. In this process, L-ascorbic acid was oxidized to dehydroascorbic acid (DHAA) by some of Fe 3+ , which were reduced to Fe 2+ . Formation process of USIO/G is schematically shown in Fig. 9. The Fe3 O4 nanoparticles with uniform distribution, which are beneficial for electrical conductivity of graphene, mitigation of volume expansion of Fe3 O4 , and facilitating Fe3 O4 particles into the electrolyte.
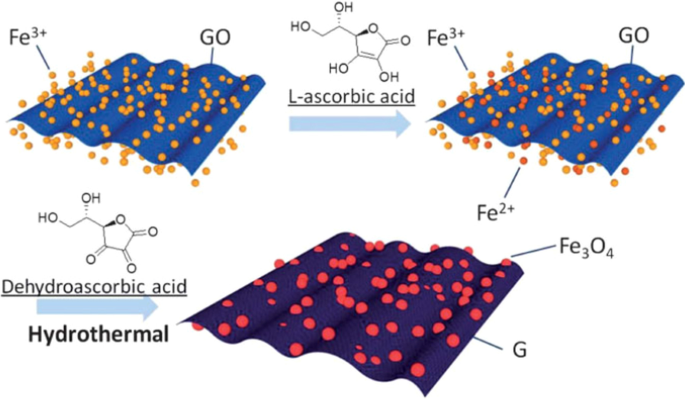
Reprinted with Permission from [127]. Copyright, Royal Society of Chemistry
Schematic illustration of the formation process of USIO/G.
Xiong et al. [128] a kind of hierarchical hollow Fe3 O4 (H-Fe3 O4 ) microspheres prepared by controlled thermal decomposition of iron alkoxide precursor. In a classical reaction, ethylene glycol (EG) serves as reduction reagent that partly reduces Fe 3+ to Fe 2+ with sodium acetate (NaAc), and polyvinylpyrrolidone (PVP) [128]. For this synthesis, PVP served as a surface stabilizer, which has important role in the formation and transformation of hollow interiors.
With the development of solvothermal synthesis, it emerging as an efficient method with the advantages of low energy consumption, little reunion and easy to control shape, etc. Chen et al. [129] synthesized poly (acrylic acid) (PAA)-entangled Fe3 O4 nanospheres by a facile solvothermal method. In their synthesis, the ethylenediamine is crucial to the controlling of the uniformity of nanospheres, and the PAA molecules served as carbon source that transforms into the carbon matrix by heating treatment in inert atmosphere. As shown in SEM image of the prepared C-Fe3 O4 nanospheres, very uniform spherical particles with a diameter of 150–200 nm are synthesized. Observed from SEM images in Fig. 10a, the nanospheres contain small irregular particles, and have a relatively rough surface. In the control experiment without ethylenediamine (EDA), the synthesized particles are much less uniform with a wider size distribution of 100–500 nm, allowing the formation of nanospheres with smaller size.
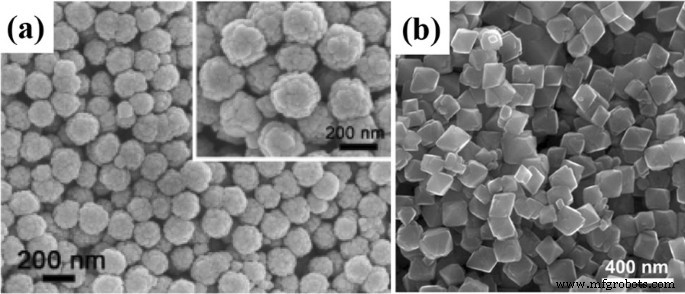
Reprinted with Permission from [130]. Copyright, Wiley–VCH
een SEM images of the Fe3 O4 nanospheres synthesized with ethylenediamine (EDA). The inset of (a ) shows a SEM image with higher magnification. (Reprinted with Permission from [129]. Copyright, American Chemical Society.). Physicochemical characterization of the octahedral Fe3 O4 nanodeeltjes. b SEM image showing the octahedral geometry of the iron oxide nanoparticles.
Co-precipitation
Due to its high cost-effectiveness, environmental friendliness, and facile synthesizing protocol, co-precipitation is a general approach for Fe3 O4 nanodeeltjes. Thus, in iron based rechargeable battery systems, Fe3 O4 nanomaterials are especially suitable for large-scale electrochemical applications to solve the energy requirement of the modern society.
Li et al. [121] proposed Fe3 O4 polyhedron as LIBs anode for alkaline secondary batteries by a co-precipitation. Annealing temperature makes a high effect on the physical and electrochemical performance of Fe3 O4 nanomaterials. The 700 °C-annealed Fe3 O4 exhibited a higher electrochemical performance, such as a higher specific discharge capacity of 604.2 mA h g −1 with a charging efficiency of 83.9% at 120 mA g −1 . Ooi et al. [130] demonstrated octahedral Fe3 O4 nanoparticles using a facile solvothermal route. Scanning electron microscope (SEM) image of Fe3 O4 nanoparticles is shown in Fig. 10b, which depicts that octahedral Fe3 O4 nanoparticles with an average length of 93 ± 18 nm were prepared by the hydrothermal method, showing a roughly Gaussian size distribution. Then, the crystal structure of octahedral nanoparticles can be further evaluated by HRTEM, and the composition of the bulk sample was further characterized by XRD and X-ray photo electron spectroscopy (XPS).
Thermolysis
The thermolysis is small monodisperse magnetic nanocrystals synthesized by organic metal compounds in high boiling point solvents containing stabilizing agent. Previous Organic metal bodies include metal acetylacetone compounds, metal cupferron, or metal Carbonyl compounds, and usually choose fatty acids, oleic acid, or hexadecyl amine as a surfactant. Zhang et al. [120] reported ultrafine Fe3 O4 nanocrystals uniformly encapsulated in two-dimensional (2D) carbon nanonetworks through thermolysis of Fe(C5 H7 O2 )3 precursor at 350 °C under vacuum, which named as 2D Fe3 O4 /C nanonetworks. This facile process using low-cost precursor proposed a green approach for preparing Fe3 O4 /carbon composite. Additionally, compared with the reported Fe3 O4 /carbon composites, the particle size of Fe3 O4 is controllable and a size of ∼ 3 nm can be obtained.
Benefitting from synergistic effects of carbon nanonetworks with excellent electrical conductivity and ultrafine Fe3 O4 particles with uniform distribution, high reversible capacity, excellent rate capability and superior cyclability at the voltage of 0.01–3.0 V (vs. Li/Li + ) are obtained. Nanoparticles with unique iron oxide (Fe3 O4 ) cores and zinc oxide (ZnO) shells were prepared by Jaramillo et al. [131]. Fe3 O4 nanoparticle synthesized through a thermolysis method using Fe(C5 H7 O2 )3 as organic metal body presoma, triethylene glycol as surface active agent, and core–shell Fe3 O4 @ZnO nanoparticles were successfully synthesized using straightforward methodologies. The structural and optical properties of the materials were characterized using a combination of X-ray diffraction, electron microscopy, and light spectroscopy. Importantly, the purity of the core and shell phases in the Fe3 O4 @ZnO nanoparticles was confirmed by both XRD and TEM, and the ZnO shell was shown to increase the transparency of the core–shell nanoparticles relative to the single-component Fe3 O4 nanodeeltjes. Zhang et al. [132] demonstrated a high crystalline Fe3 O4 -graphene composite by one-step reaction of thermolysis. And they demonstrated that the attachment of iron-organic complex with graphene oxide (GO) sheets can facilely result in magnetic graphene composites via a time-dependent calcination process.
Sol–Gel Process
The specific method is using the metal alkoxide, metal mineral compound or a mixture of the above two substances to hydrolysis and polymerization, uniform gel gradually, then condense into a transparent gel, however, after drying and heating, finally the oxide ultrafine powders was received. Tang et al. [122] prepared nanostructured magnetite thin film by sol–gel method using inexpensive iron (II) chloride precursor. Fe3 O4 nanoparticles were prepared at 300 °C, however, α-Fe2 O3 is generated when temperature increased to 350 °C, and this result restricts its applications. Xu et al. [123] proposed magnetite nanoparticles by virtue of sol–gel process combined with annealing in vacuum at 200–400 °C using nontoxic and low-cost ferric nitrate. In their study, Fe3 O4 nanoparticles with various sizes can be synthesized facilely through varying the annealing temperature.
Micro-emulsion Method
Micro-emulsion is composed of two mutual miscibility of liquid mixture of thermodynamic stability and isotropy dispersion, one of these or two kinds of liquid called micro area, and fixed by interface layer of the surfactant molecules. The key factors controlling the reaction solution contain concentration, pH value, reaction time and temperature. Micro-emulsion as a rapid expansion of new technology possesses many advantages. For example, high purity and uniform particle size distribution molecular dopant was synthesized at low temperature and simple reaction process. But there are also some shortcomings, for instance, the reaction system mostly contains organic solvents, which leads to high cost, pollution of environmental health and long reaction time. The prepared Fe3 O4 nanoparticles have excellent catalytic performance for the synthesis of quinoxaline in different solvents. Novel core–shell magnetic Fe3 O4 /silica nanocomposites with triblock-copolymer grafted on their surface (Fe3 O4 @SiO2 @MDN) were successfully synthesized by combining sol–gel process with seeded aqueous-phase radical copolymerization approach [133]. The Fe3 O4 @SiO2 @MDN microspheres were synthesized in following three steps. Firstly, the initial magnetic Fe3 O4 microspheres were synthesized by a solvothermal reaction. Then a sol–gel process was utilized to prepare silica coated Fe3 O4 microspheres (Fe3 O4 @SiO2 ), and a thin amorphous silica layer was formed on Fe3 O4 microspheres. Afterward, the Fe3 O4 @SiO2 microspheres were modified by 3-(methacryloxypropyl) trimethoxysilane (MPS). Finally, the triblock copolymer was fabricated by aqueous phase radical copolymerization reaction among MPS, divinylbenzene (DVB) and N-Vinyl-2-pyrrolidone (NVP) on the surface of Fe3 O4 @SiO2 . The magnetic Fe3 O4 particles with narrow size distribution have nearly spherical shape and smooth surface. Li et al. [124] reported hexagonal and triangular monodisperse Fe3 O4 nanosheets by a two-step microemulsion solvothermal approach, in which the uniform Fe3 O4 nanoparticles are prepared and then these hydrophobic nanocrystals are dispersed in a uniform microemulsion environment as “seeds” for further re-growth through a secondary solvothermal process. In the first step, near-spherical monodisperse 7–8 nm Fe3 O4 nanoparticles were formed through a kinetically controlled process. In the second step, the formation of anisotropic Fe3 O4 nanosheets is a thermodynamically controlled process and all the exposed surfaces of the triangular and hexagonal nanosheets are (111) crystal planes, which have the lowest surface energy for FCC Fe3 O4 .
Other Methods
Physical methods are also significant ways to prepared Fe3 O4 nanostructure for anode of LIBs. Several advantages, such as good crystallization, fine-tuned particle size, and high purity of products are highlighted in recent literatures. But these methods usually demand advanced and expensive equipment, result in a higher cost, poor dispensability of particles dispersion, and agglomeration of nanostructures. For instance, Du et al. [109] fabricated activated carbon (AC)-Fe3 O4 nanoparticles asymmetric supercapacitor, and Fe3 O4 nanostructure was prepared by microwave method. The precursor, FeSO4 ·7H2 O and NH3 ·H2 O mixed solution, was heated in microwave oven. The black precipitate was separated by magnet and washed repeatedly with DI water. The resulted microstructural properties of prepared nanoparticle were characterized by nitrogen adsorption (Quantachrome NOVA 2000), XRD and SEM [109]. Chen et al. [127] synthesized graphene nanosheets decorated with ultra-small Fe3 O4 nanoparticles (USIO/G). Seo et al. [134] reported an integrated usage of magnetic particles in microalgal downstream processes, specifically microalgal harvesting and lipid extraction through one-step aerosol spray pyrolysis and applied in microalgal harvesting and serial microalgal lipid entrapment. TEM/EDS, XPS, and FT-IR analysis suggested that the cationic and lipophilic functionalities arose from not fully decomposed PVP, due to the short residence time in the reactor. Kang et al. [135] proposed Fe3 O4 nanocrystals confined in mesocellular carbon foam (MSU-F–C) by a “host–guest” approach and applied it as LIBs anode. In this study, a precursor of Fe(NO3 )3 ·9H2 O is impregnated in MSU-F–C having uniform cellular pores with a diamter of ~ 30 nm, followed by heating treatment at 400 °C for 4 h in argon (Ar) atmosphere. Fe3 O4 nanocrystals with size of 13–27 nm were fabricated inside the pores of MSU-F–C. The existance of the carbon most likely allows the reduction of some Fe 3+ to Fe 2+ ions by a carbothermoreduction process. The physical performance and pore structure of MSU-F–C and Fe3 O4 -loaded composites were characterized with nitrogen sorption, and the composites have high capacities of ∼800–1000 mA h g −1 at 0.1 A g −1 (∼0.1 C rate), high rate capability and good cycling performance.
Application
Fe3 O4 possesses lots of unique properties, and is highly promising for applications in LIBs and supercapacitors [136,137,138,139,140,141,142]. Table 4 summarizes some applications.
Li-Ion Batteries
Due to conversion reaction of Fe3 O4 during charge/discharge process and other advantages, the Fe3 O4 is usually studied and applied as LIBs anode [143,144,145,146,147]. For TMOs, they have higher theoretical capacity (~ 500–1000 mA h g −1 ) than conventional graphite (about 372 mA h g −1 ). Furthermore, Fe3 O4 has superior conductivity compared with other transition metal oxides. Thus, it is well-focused by recent studies. It has been reported that composite electrodes with graphene have high performance due to their large surface area, high electrical conductivity and adaptive or flexible structure for high reliability. Qiu et al. [3] reported a kind of composite anode composed of ultra-dispersed Fe3 O4 nanoparticles (3–8 nm) and RGO sheet. It has excellent cyclic performance (624 mA h g −1 for up to 50 charge/discharge cycles at a current density of 0.1 A g −1 ), and good specific capability (624 and 415 mAh g −1 at 0.1 and 2.4 A g −1 , respectively) for LIBs. The obtained Fe3 O4 /RGO exhibited high and ultrastable Photo-Fenton activity (Fig. 11).
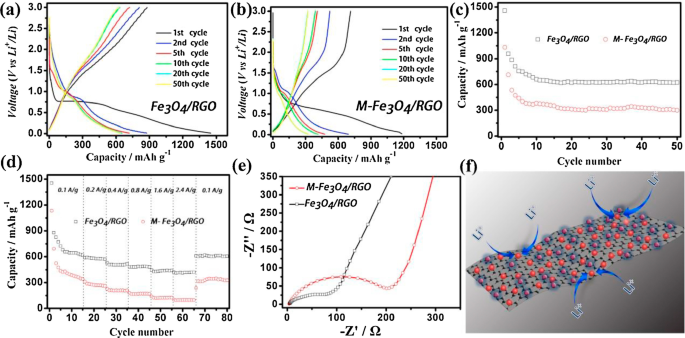
Reprinted with Permission from [3]. Copyright, Elsevier B.V
The charge/discharge curve of Fe3 O4 /RGO composites (a ) and mechanically mixed Fe3 O4 /RGO composites (M-Fe3 O4 /RGO) (b ) electrodes at constant current densities of 0.1 A g −1 . Cycling performance of Fe3 O4 /RGO composites and M-Fe3 O4 /RGO composites electrode at constant current densities of 0.1 A g −1 (c ). Rate capability of Fe3 O4/ RGO composites and physically mixed Fe3 O4 /RGO composites at the current densities between 0.1 A g −1 and 2.4 A g −1 (d ). Nyquist plots of the electrodes of Fe3 O4 /RGO sheet and mechanically mixed Fe3 O4 /RGO composites. All of the measurements were conducted using a voltage window of 0.01–3.0 V (e ). Schematic representation of the electrochemical reaction path on the Fe3 O4 /RGO composites (f ).
Pyrolyzed carbon is also a good “companion” for Fe3 O4 anodes. Apart from the facile protocol, porous structure formed by pyrolysis always exhibits high specific capacity of Fe3 O4 composite anode. Wang et al. [12] reported hollow N-doped Fe3 O4 /C nanocages with hierarchical porosities by carbonizing polydopamine-coated PB nanocrystals as LIBs anode (Fig. 12). The specific capacity of N-doped Fe3 O4 /C nanocages is ~ 878.7 mA h g −1 after 200 cycles at a current density of 200 mA g −1 , much higher than that of N-doped Fe3 O4 /C derived from pure PB (merely 547 mA h g −1 ). It is also desirable to design anisotropic structure of Fe3 O4 nanoparticles with carbon coated layer. Zhang et al. [19] reported a kind of carbon-coated Fe3 O4 nanospindles derived from α-Fe2 O3 nanospindles with length of about 500 nm and an axis ratio of ~ 4. Following by a hydrothermal synthesis method with glucose, the obtained LIBs anode delivered a high reversible capacity of ~ 745 mA h g −1 at C/5 and ~ 600 mA h g −1 at C/2.
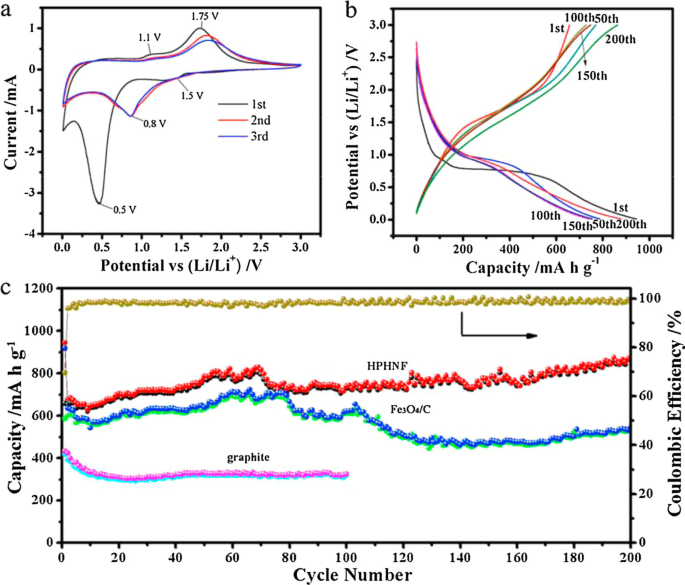
Reprinted with Permission from [12], Copyright, Elsevier Ltd
een CVs of the HPHNF during the first three cycles at 0.2 mV s −1 , b Galvanostatic charge/discharge profiles of the HPHNF electrodes for the 1st, 50th, 100th, 150th and 200th cycle at a specific current of 200 mA g −1 . c Cycling performance of the HPHNF nanocomposites, N-doped Fe3 O4 /C nanocomposites and graphite at a specific current of 200 mA g −1 . d Coulombic efficiency of HPHNF.
The most impressive work towards this field is probably the mesoporous iron oxide nanoparticle clusters with carbon coating reported by Lee et al. [148]. After a few cycles, the formation of SEI greatly enhanced the stability of interface between electrode and electrolyte. Electrochemical test exhibited a high specific capacity of 970 mA h g −1 for LIBs.
Supercapacitors
Fe3 O4 is a highly promising candidate for supercapacitor electrode because of its relatively high electrical conductivity, fast reversible redox reaction, low cost and eco-friendly nature [149,150,151,152]. Similar to batteries, high performance supercapacitors also require two factors:large specific surface area and long-term stability. Those two features usually were achieved by building some porous structures and carbon coated layers. Fe3 O4 nanoparticle with a high specific surface area was synthesized by Wang et al. [126] using a bottom up approach. Ferric chloride was firstly sonicated with ethanolamine and then processed through a solvothermal reaction. The obtained active nanomaterials showed a specific surface area of 165.05 m 2 g −1 and a specific capacitance of 207.7 F g −1 at 0.4 A g −1 .
Also, highly dispersed Fe3 O4 nanosheets on 1D CNFs is reported by Mu et al. [125]. The Fe3 O4 /CNFs composites showed a higher specific capacitance than pure Fe3 O4 in 1 M Na2 SO3 . To further enlarge the specific capacitance and cycle stability, hierarchically porous carbon spheres with Fe3 O4 using as supercapacitors exhibited high capacitivity of 1153 F g −1 at 2 A g −1 and high specific capacitance of 514 F g −1 at 100 A g −1 . In addition, the assembled asymmetric supercapacitor with double-shelled hollow carbon spheres and Fe3 O4 , has excellent cycling stability (96.7% retention after 8000 cycles) and high energy density (17–45 Wh kg −1 ) at a power density of 400–8000 W kg −1 [4].
Conclusion
Iron oxides (Fe1−x O, Fe2 O3 , Fe3 O4 ) based nanostructures have much higher specific capacities than those of commercial carbon based anodes. They are considered as highly promising candidates for LIBs anode. However, large irreversible capacity and low cycle stability are two serious problems that obstruct the application of iron oxides based nanostructures. In this review, we summarized the recent progress on novel iron oxides and their composites as LIBs anode and supercapacitor electrode. Several typical synthetic methods of various novel iron oxides based nanostructures are listed. By comparing the electrochemical performance of these various iron oxides based nanostructures, some strategies are expected to solve the problems of iron oxides based nanostructures.
Beschikbaarheid van gegevens en materialen
Niet van toepassing.
Nanomaterialen
- Cervoz:de juiste flash-opslag kiezen voor industriële toepassingen
- AI optimaliseren voor embedded applicaties
- PPA voor e-mobiliteitstoepassingen
- Drie grote vragen voor iedereen die de arena voor energieopslag betreedt
- Nanodeeltjes voor kankertherapie:huidige vooruitgang en uitdagingen
- Op cellen gebaseerde medicijnafgifte voor kankertoepassingen
- Vooruitgang in op ijzeroxide gebaseerde nanostructuren voor toepassingen in energieopslag
- Schatting van supercapacitor-energieopslag op basis van fractionele differentiaalvergelijkingen
- Evaluatie van grafeen/WO3 en grafeen/CeO x-structuren als elektroden voor supercondensatortoepassingen
- Oppervlaktenanostructuren gevormd door fasescheiding van metaalzout-polymeer nanocomposietfilm voor antireflectie en superhydrofobe toepassingen
- Vraag en antwoord:olie- en gasbronnen voor energieopslag