Boorkoolstofoxynitride als een nieuwe metaalvrije fotokatalysator
Abstract
Op boor gebaseerde nanomaterialen zijn in opkomst als niet-toxische, aardovervloedige (foto)elektrokatalysatormaterialen in zonne-energieconversie voor de productie van zonne-waterstofbrandstof en milieusanering. Boorkoolstofoxynitride (BCNO) is een quaternaire halfgeleider met elektronische, optische en fysisch-chemische eigenschappen die kunnen worden aangepast door de samenstelling van boor, stikstof, koolstof en zuurstof te variëren. De relatie tussen de structuur van BCNO en de fotokatalytische activiteitsrelatie moet echter nog worden onderzocht. We hebben een diepgaande spectroscopische analyse uitgevoerd om het effect van het gebruik van twee verschillende stikstofprecursoren en het effect van gloeitemperaturen bij de bereiding van BCNO op te helderen. BCNO-nanoschijven (D =-6,7 ± 1,1 nm) met turbostratische boornitride-diffractiepatronen werden bereid met gebruikmaking van guanidinehydrochloride als de stikstofbronvoorloper na thermisch uitgloeien bij 800°C. De röntgenfoto-elektronspectroscopie (XPS) oppervlakte-elementanalyse van de BCNO-nanoschijven onthulde dat de B-, C-, N- en O-samenstellingen respectievelijk 40,6%, 7,95%, 37,7% en 13,8% waren. Volgens de solid-state 11 B NMR-analyses, de van guanidinehydrochloride afgeleide BCNO-nanoschijven toonden de vorming van verschillende driecoördinaat BNx (OH)3−x soorten, die ook dienden als een van de fotokatalytische actieve plaatsen. De XRD en diepgaande spectroscopische analyses bevestigden de voorbereiding van BCNO-gedoteerde hexagonale boornitride-nanoschijven. Daarentegen bestond de BCNO, gegloeid bij 600 ° C met behulp van melamine als stikstofvoorloper, uit gelaagde nanosheets bestaande uit B-, C-, N- en O-atomen die covalent waren gebonden in een honingraatrooster als bewijs door de XRD, XPS en vaste toestand NMR analyse ( 11 B en 13 C) analyses. De XPS-oppervlakte-elementsamenstelling van de van melamine afgeleide BCNO-gelaagde structuren bestond uit een hoge koolstofsamenstelling (75,1%) met een relatief lage boor (5,24%) en stikstof (7,27%) samenstelling, wat wijst op de vorming van met BCNO gedoteerde grafeenoxiden gelaagde plaatstructuren. Deze reeks van melamine-afgeleide BCNO-gedoteerde grafeenoxide gelaagde structuren bleken de hoogste fotokatalytische activiteit te vertonen, die de fotokatalytische activiteit van grafietkoolstofnitride overtreft. In deze gelaagde structuur is de vorming van de tetracoördinaat BNx (OH)3−x (CO) soorten en de rijke grafietdomeinen werden voorgesteld om een belangrijke rol te spelen in de fotokatalytische activiteit van de BCNO-gedoteerde grafeenoxiden gelaagde structuren. De optische bandgap-energieën werden gemeten als 5,7 eV en 4,2 eV voor respectievelijk BCNO-gedoteerde hexagonale boornitride-nanoschijven en BCNO-gedoteerde grafeenoxiden-gelaagde structuren. Ten slotte vertoonde BCNO een ultralange fotoluminescentie met een gemiddelde vervallevensduur van respectievelijk 1,58, 2,10, 5,18 en 8,14 µs voor BGH01, BGH03, BMH01, BMH03. Deze studie biedt een nieuw metaalvrij fotokatalytisch systeem en biedt de eerste structurele analyse met betrekking tot de oorsprong van op BCNO gebaseerde fotokatalysatoren.
Grafische samenvatting
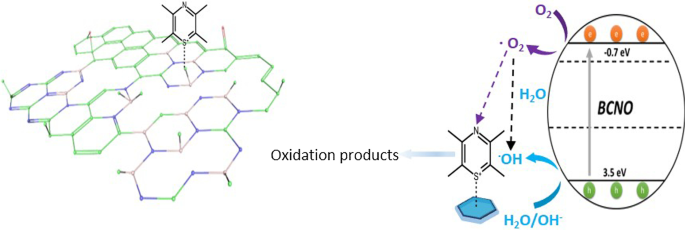
Inleiding
Metaalvrije nanomaterialen zijn in opkomst als kosteneffectieve, milieuvriendelijke (foto)katalysator met hoge structurele en chemische stabiliteit voor verschillende toepassingen, waaronder de productie van zonnebrandstof, milieusanering, CO2 vermindering, desinfectie van schadelijke micro-organismen en maakte selectieve chemische synthese van organische verbindingen mogelijk [1,2,3,4,5,6,7]. Vergeleken met hun metalen tegenhangers is een metaalvrije katalysator ook minder vatbaar voor vergiftiging en leidt tot een langere levensduur van de cyclus. Het zoeken naar en ontwikkelen van nieuwe materialen die stabiele, efficiënte en kosteneffectieve fotokatalysatorresten zijn, zijn dus cruciale en uitdagende onderzoeksinspanningen. Op koolstof gebaseerde materialen zoals grafietkoolstofnitride (CN) [4, 8, 9], carbon-dot (C-dot) [2, 3, 10] en op grafeen gebaseerde materialen [7, 11] zijn uitgebreid onderzocht vanwege hun uitstekende fysisch-chemische eigenschappen, structurele en chemische stabiliteiten en het gemak van synthese uit aardrijke elementen. Onlangs zijn op boor gebaseerde (foto)katalysatoren ontwikkeld als metaalvrije fotokatalytische systemen met opmerkelijke prestaties. Met name boorcarbide, bekend om zijn hardheid, vertoonde metaalvrije zichtbaar licht fotokatalytische waterstofgeneratie, wat de ultramoderne op koolstof gebaseerde CN-fotokatalysator overtreft [12, 13]. Met koolstof gedoteerde hexagonale boornitride (BCN) nanoplaten met groot oppervlak die zichtbaar licht fotokatalytische activiteit vertonen voor H2 enO2 generatie en CO2 reductie en vastleggen hebben geleid tot nieuwe mogelijkheden in het fotosysteem [14, 15]. Andere boorhoudende (foto)elektrokatalysatoren zoals booroxynitride (BNO)[16, 17], boorfosfide (BP) [18, 19], met boor gedoteerd grafeen [20], boorkoolstofnitride (BCN) [14], met boor gedoteerd koolstofnitride (B-gedoteerdCN ) [21] en elementair boor [22, 23] hebben significante (foto) elektrokatalytische activiteiten [13] aangetoond.
Boorkoolstofoxynitride (BCNO) is een op boor gebaseerd nanomateriaal dat minder is bestudeerd dan andere materialen. Het werd voor het eerst ontwikkeld na hun BCN-voorganger, een halfgeleider met een bandafstand van ongeveer -2 eV, ter vervanging van giftige fosforen op basis van oxynitride en nitrideverbindingen [24, 25]. De substitutie van B-, C-, O- en N-atoom in het grafeen- of hexagonaal boornitride (hBN) -netwerk leidde tot BCNO-verbindingen met afstembare fotoluminescentie-eigenschappen en een bandgap variërend van 0 eV (grafeen) tot 5,9 eV (hBN) [26] . Deze gewenste halfgeleider- en fotoluminescentie-eigenschappen hebben onlangs onderzoekers aangetrokken om een nieuwe synthetische methodologie te ontwikkelen voor het synthetiseren van laagdimensionale BCNO-nanostructuren met hogere kristalliniteit [27], gecontroleerde vormen [28] en in atomair dunne 2D-structuren [29]. Eerdere werken onderzochten de effecten van uitgloeitemperaturen en -tijden bij het moduleren van de fotoluminescentie-eigenschap van BCNO zonder diepgaande structurele karakterisering te bieden [30, 31]. In dit artikel hebben we het effect onderzocht van het gebruik van verschillende voorlopers van stikstofbronnen, calcineringstemperaturen (800 °C vs. 600C) en calcineringstijden (0,5 uur vs. 12 uur) op de structuur-fotokatalytische activiteit van BCNO-nanostructuren.
Methoden
Chemische stoffen en instrumenten
Boorzuur 99,99% (H3 BO3 ), melamine 99% (C3 H6 N6 ), en hexamethyleentetramine ≥ 99% (C6 H12 N4 ) werden gekocht bij Alfa Aesar en zonder verdere zuivering gebruikt. Guanidinehydrochloride 99,5% (CH5 N3 HCl) werd gekocht bij Arcos Organics. BCNO werd volgens de literatuur gesynthetiseerd via de lage-temperatuur-gloeimethode [25, 32]. UPS-analyse werd uitgevoerd in een ULVAC-PHI PHI 5000 Versaprobe II met He I 21,22 eV als een fotonenbron met een bias van 5 V. De morfologie van BCNO-monsters werd geanalyseerd via een transmissie-elektronenmicroscoop (JEOL, JEM-ARM200FTH). XRD-diffracties werden verkregen met behulp van de Bruker D2-spectrometer. Fotoluminescentie-emissiespectra in oplossing werden verkregen met behulp van een fotoluminescentiespectrometer (PerkinElmer, LS55), en optische absorptiespectra van BCNO in oplossing werden bepaald door de UV-Vis-spectrometer (HITACHI, U-3900). Röntgenfoto-elektronspectroscopie werd geanalyseerd via röntgenfoto-elektronspectrometer met hoge resolutie (ULVAC-PHI, PHI Quantera II) met behulp van Al Ka-röntgenstralen als de excitatiebron. De BCNO-oplossing werd druppelgegoten op het siliciumsubstraat voor XPS-karakterisering. De bindingsenergie werd gekalibreerd tot koolstof bij 284,8 eV. XPS-piekdeconvolutie en aanpassing werden uitgevoerd met behulp van CACS XPS-software. Absolute PLQY werd uitgevoerd volgens de literatuur [33] en gedetecteerd door een CCD-camera (PIXIS 256BR, Princeton Instruments). De meting van absolute PLQY werd uitgevoerd met behulp van een op vezels gebaseerde spectrograaf, inclusief een gekalibreerd bolsysteem (Labsphere) en een camera met ladingsgekoppelde apparaten (CCD) (PIXIS 256BR, Princeton Instruments). Een diodelaser (λ = 375 nm, Becker &Hickl GmbH) werd gebruikt als pompbron. Tijdsopgeloste fotoluminescentie werd gemeten in een front-face configuratie met behulp van een gepulseerde stikstoflaser (λ = 337.1 nm, LTB Lasertechnik Berlin GmbH) als een excitatiebron, die werd geactiveerd door een digitale vertragingsgenerator (DG645, Stanford Research Systems). De signalen werden gedetecteerd door een fotonentellende fotomultiplicatorbuis (PMC-100-1, Becker &Hickl GmbH) en de fotonentellingen werden verzameld met een multiscalermodule (MSA-300, Becker &Hickl GmbH). Infraroodspectra werden geregistreerd door Fourier-transform infraroodspectrometer (Bruker, Vertex 80v) met behulp van Attenuated Total Reflectance (ATR). Nucleaire magnetische resonantiespectra werden verkregen met behulp van Bruker Avance III 400 NMR-spectrometer uitgerust met een 9,4 T-magneet met behulp van een 4 mm magische hoekspin (MAS) -sonde. 11 B MAS NMR werden geregistreerd met behulp van de spin-echo-methode met een spinsnelheid van 10 kHz. Er werden 8000 scans verzameld met een recyclevertraging van 4 s. Chemische verschuivingen werden verwezen naar 1 M H3 BO3 waterige oplossing bij 19,6 ppm. 13 C CP/MAS NMR-spectra werden opgenomen met behulp van een kruispolarisatie (CP) -sequentie met een spinsnelheid van 12,5 kHz. Er werden 30.000 scans verzameld met een recyclevertraging van 4 s. Alle 13 C chemische verschuivingen werden verwezen naar puur trimethylsilaan met behulp van de secundaire referentie van het adamantaan CH2 piek bij 38,48 ppm.
Voorbereiding van BCNO
In deze studie werden twee reeksen BCNO bereid met behulp van twee verschillende stikstofprecursorbronnen terwijl de boor- en koolstofprecursorbronnen, precursorverhoudingen, gloeitemperatuur en tijd werden gefixeerd. Het effect van gloeitemperaturen en -tijden werd ook onderzocht door alle andere reactieparameters vast te stellen voor elke reeks BCNO die in dit onderzoek werd bereid. In het kort werden alle drie de voorlopercomponenten in een vooraf bepaalde molverhouding toegevoegd aan gedestilleerd water en verwarmd tot 90 ° C totdat de oplossing homogeen leek (tabel 1). Het kleverige mengsel werd een nacht in een oven gedroogd, wat een gedroogde witte vaste stof opleverde. De witte vaste stof werd vermalen met behulp van een vijzel en stamper tot fijne poeders. De vaste voorloper werd in de oven gecalcineerd bij een vooraf bepaalde temperatuur en tijd, zoals weergegeven in tabel 1, met een hellingssnelheid van 5 °C/min onder atmosferische omgevingsdruk. Het geelachtig getinte poedermonster werd vermalen tot een fijn poeder nadat de oven op natuurlijke wijze was afgekoeld tot kamertemperatuur.
Zuiveringsstrategieën van BCNO
De bereide BCNO werd gezuiverd door 10 min centrifugeren bij 6000 rpm in water en ethanol (1:10 v/v in een concentratie van 10 mg/ml). Na centrifugeren werd het product opnieuw opgelost in gedestilleerd water, verdund met ethanol in een 1:10 v/v verhouding van water:ethanol. Het gezuiverde BCNO werd voor TEM-analyse op een met koolstof gecoat koperen rooster afgezet. Voor de bereiding van SEM- en XPS-monsters werd het gezuiverde BCNO-monster op een siliciumwafel gegoten. Voorafgaand aan monsterafzetting werden siliciumwafels gereinigd door sonicatie met water, propanol en aceton gedurende 10 minuten in elk oplosmiddel. Het UPS-monster werd op dezelfde manier bereid als de procedure die is beschreven voor SEM- en XPS-monsters, behalve dat de monsters werden afgezet op een met indiumtinoxide (ITO) gecoat glas.
Bereiding van bulkkoolstofnitride (CN)
Bulk-CN werd gesynthetiseerd met een gemakkelijke methode die in de literatuur wordt vermeld [34]. In het kort, melaminepoeder werd in een smeltkroes geplaatst en gedurende vier uur bij 550 gegloeid met een hellingssnelheid van 5 ℃ / min onder atmosferische omgevingsdruk.
Procedure voor fotokatalytische kleurafbraak
De fotokatalytische activiteiten van verschillende BCNO-monsters werden geëvalueerd via de methyleenblauw (MB) fotodegradatie als een modelreactie. In een typisch kleurstofafbraakexperiment werd 10 mg BCNO-monster toegevoegd aan een monsterflesje met 15 ml MB-oplossing (10 ppm). Na 10 minuten roeren in het donker werd het monsterflesje bestraald met een 100 W Xenonlamp (250 nm ~ 1100 nm). Kinetische monsters (2 ml) werden met een pipet uit de oplossing geëxtraheerd met een tijdsinterval van 20 minuten totdat de totale fotokatalytische afbraaktijd 80 minuten bereikte. Kinetische monsters op verschillende tijdsintervallen werden geanalyseerd via een UV-vis-spectrometer. De verandering in de concentratie van MB werd afgeleid met behulp van de wet van Beer.
Resultaten en discussie
Synthese van BCNO
In ons onderzoek voor de bereiding van laagdimensionale BCNO-nanostructuren met een hoge kristalliniteit om ladingstransport te vergemakkelijken, hebben we BCNO ontdekt met duidelijk verschillende chemische structuren en fotokatalytische activiteiten. Op basis van de synthese van BCNO zoals gerapporteerd in de literatuur [25, 32], hebben we het effect onderzocht van het gebruik van twee verschillende stikstofprecursorbronnen, evenals het effect van thermische gloeitemperaturen en tijd op de structuur-eigenschapsevolutie van BCNO. In deze studie werden twee series BCNO bereid met respectievelijk boorzuur en hexamethyleentetramine als boor- en koolstofbronnen (tabel 1). De BCNO werd gesynthetiseerd met melamine en guanidinehydrochloride als stikstofbron en wordt respectievelijk aangeduid als BMH en BGH. Na systematisch elke reactieconditie te hebben onderzocht, vertoonde de BMH-serie alleen fotokatalytische activiteiten wanneer ze gedurende 12 uur bij de lagere temperatuur van 600 ° C werden uitgegloeid. De BGH-serie vertoonde alleen fotokatalytische activiteiten met gloeien bij hoge temperatuur bij 800 ° C gedurende 12 uur. In beide reeksen werden de molverhoudingen van boorzuur, melamine en guanidinehydrochloride vastgesteld op 3:1, terwijl de molverhoudingen van hexamethyleentetramine werden gevarieerd van 0,1 tot 0,3. Deze ratiso van hexamethyleentetramine-precursor wordt in de namen van de monsters aangegeven als respectievelijk BMHH01/BGH01 en BMH03/BGH03 (tabel 1).
Afbeelding 1 toont de representatieve TEM-beelden van BGH gecalcineerd bij 800 °C gedurende 12 uur, samengesteld uit een kristallijne kern met quasi-bolvormige nanodeeltjesmorfologie en D = 6.7 ± 1 nm. Het zoals bereide BGH werd gemakkelijk gedispergeerd in water (aanvullend bestand 1:Fig. S1), en zoals blijkt uit de afzetting van discrete nanodeeltjes op het TEM-koperrooster. De dispergeerbaarheid van BGH en BMH in water is hoogstwaarschijnlijk afkomstig van elektrostatische stabilisatie op basis van hun negatief geladen oppervlaktepotentiaal van BGH en BMH. Halfgeleider 11 B NMR-analyses onthulden dat de overvloed aan hydroxylgroepen in zowel BMH- als BGH-nanostructuren hun dispergeerbaarheid in waterige media bevestigde. Onder hoge vergroting vertoonde elk quasi-sferisch nanodeeltje een onderscheidende roosterafstand van de (002) vlakken, gemeten als 0,338 nm, wat consistent is met literatuurrapporten [24, 35] (Fig. 1c). Vergeleken met het 5 nm BCNO-nanodeeltje verkregen door thermisch gloeien in de eutectische zoutomgeving, bezaten de BGH-nanodeeltjes die in dit werk werden bereid een hoge kristalliniteit [25] (Fig. 1). Vervolgens karakteriseerden we BMH dat was opgesteld op basis van een literatuurrapport met boorzuur, melamine en hexamethyleentetramine en gedurende 12 uur bij 600 ° C was gegloeid. In tegenstelling tot de morfologie van BGH, is BMH samengesteld uit meerlagige vellen met slecht gedefinieerde vormen (Fig. 1). Bij hogere vergroting onthulde het TEM-beeld van de randen op de meerlagige vellen (figuur 1d) nanovellen met kenmerken van structurele vervorming [36] zoals weergegeven in figuur 1e. Figuur 1f toont een representatief SEM-beeld van de BMH inclusief kenmerkende aggregaten van micronformaat. In tegenstelling tot de BGH-serie leverde calcineren bij hogere temperatuur bij 800 ° C met melamine als de stikstofbronvoorloper echter geen nanodisk-morfologie op. De TEM, XRD, UV-absorptie en fotoluminescentie van de andere verbindingen uit de BGH- en BMH-reeks die bij verschillende reactieomstandigheden zijn gesynthetiseerd, zijn beschikbaar in de ESI.
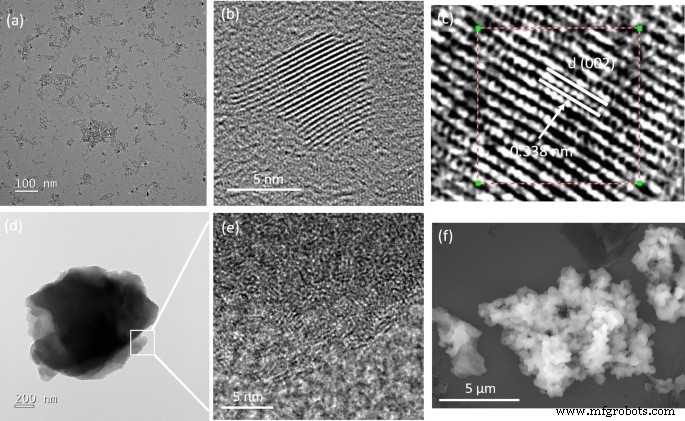
Representatieve transmissie-elektronenmicroscoop (TEM) en scanning-elektronenmicroscoop (SEM) van de bereide BGH en BMH afgezet uit een verdunde ethanoloplossing. een , b Hoge en lage vergroting TEM van BGH, c hoge resolutie TEM van BGH met duidelijke (002) roosterafstand van 0,338 nm, d TEM-beeld van representatieve BMH bij lage vergroting, e uitgebreide TEM-afbeelding van het omkaderde gebied in Fig. 1d met kenmerken van structurele vervorming binnen het gelaagde grafeenoxide, en f representatief SEM-beeld van BMH
Figuur 2 toont de XRD-patronen van BMH03 (groen spoor), BMH01 (rood spoor) en BGH01 (blauw spoor) bereid in ons laboratorium onder de voorgeschreven reactieomstandigheden zoals vermeld in Tabel 1. De BGH01 vertoonde brede diffractiepieken gecentreerd rond 26,6° en 43,1 ° (2θ), het kenmerkende diffractiepatroon van turbostratisch boornitride (t-BN). De brede piek gecentreerd op ongeveer 26,6 ° was afkomstig van het (002) reflectievlak, en de 43,1 ° brede piek komt overeen met het (10) reflectievlak geïnduceerd door hexagonaal boornitride (h-BN) [37]. Het XRD-patroon van BMH werd gedomineerd door twee brede diffractiepatronen op 2θ van ongeveer 25,4 ° en 42,4 °, die kenmerkende patronen zijn voor respectievelijk de (002) en (10) banden van de hexagonale kristalstructuur van grafiet [38]. De algemeen aangeduide (10) band wordt ook geassocieerd met de 2D-reflectie van turbostratisch koolstof [39]. Bovendien was de afwezigheid van een piek bij 2θ ongeveer 10,9° en de opkomst van een brede band bij ongeveer 25,4° te wijten aan de opname van doteerstoffen of onzuiverheden in de structuur van grafeen of grafietoxiden [40,41,42,43, 44,45]. Daarom is het redelijk om voor te stellen dat de dominante structuur van BMH gedoteerde grafeenoxiden is.
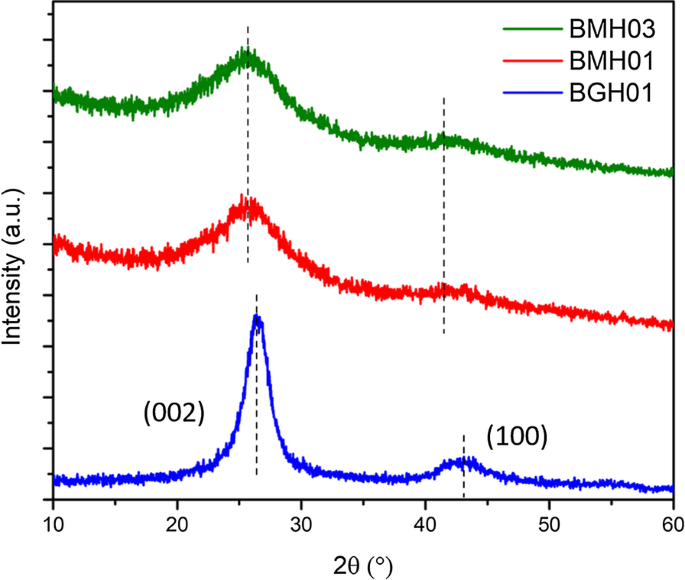
De XRD-patronen van polykristallijn BMH03 (groen spoor), BMH01 (rood spoor), BGH01 (blauw spoor) en BGH01. De brede piek van de BGH01-serie op ca. 26,6 ° vertegenwoordigt de (002) vlakreflectie van h-BN en een andere brede piek op ca. 43,1° vertegenwoordigt de onopgeloste reflectievlakken van h-BN. Diffractiepatronen van de BMH vertoonden twee brede reflecties gecentreerd rond ~ 25.4° en ~ 42.4°. De XRD-patronen van andere BCNO-series worden weergegeven in Aanvullend bestand 1:Afbeelding S3)
Structurele analyse van BGH (Guanidine-serie)
De XPS-, FTIR- en solid-state NMR-spectroscopie werden uitgevoerd om dieper inzicht te krijgen in de moleculaire structuur van BGH. De XPS werd gebruikt om de aanwezigheid van de elektronen op kernniveau van de B-, C-, N- en O-elementen en hun respectieve chemische binding in BGH-verbindingen te bevestigen. Afbeelding 3a–e toont een typisch XPS-spectra van de BGH-nanoschijven. Volgens de XPS-analyses van de oppervlakte-elementsamenstelling bevatte BGH een hoog B- en N-gehalte (elk ongeveer 40%), met een lagere C- en O-samenstelling van respectievelijk ongeveer 8% en 13% (Aanvullend bestand 1:Tabel S3). De bijna 1:1-stoichiometrie van de B- en N-samenstelling komt overeen met de XRD-analyses, die bevestigden dat BGH01, bereid in ons laboratorium, was samengesteld uit een turbostratische boornitridestructuur. Alle XPS-spectra waren uitgerust met een Gauss-functie met R 2 > 0,99, wat wordt weergegeven door de rode en groene curven in de XPS-spectra voor elk element. Voor de BGH-serie, de B1s spectra werden gedeconvolueerd in twee aangepaste curven, die overeenkwamen met BO-binding (bij 189,7 eV bindingsenergie) en BN-binding (bij 190,7 eV bindingsenergie). De N1s spectra werden uitgerust met twee Gauss-curven bestaande uit NB-binding met 397,3 eV-bindingsenergie en BN-O-binding met 398 eV-bindingsenergie [46]. Zowel N-B- als B-N-O-binding duidden op de aanwezigheid van O-gedoteerde hBN of de vorming van een BNO-verbinding [47]. Omdat de synthese van BGH werd uitgevoerd onder atmosferische omstandigheden, werd ook een zuurstofatoom opgenomen in het h-BN-domein voor B-O-binding, zoals vermeld in de literatuur [47]. Deze hypothese werd bevestigd door het analyseren van de O1s spectra, die een enkele piek vertoonden die overeenkomt met de B-O-binding (bindingsenergie bij 532 eV). Dit resultaat ondersteunt verder onze aanvankelijke hypothese dat de BGH-reeks is samengesteld uit O-gedoteerde hBN en dat het grootste deel van het zuurstofatoom is gebonden aan het booratoom. De C1s van het BGH-spectrum werd gedeconvolueerd in drie C-soorten, namelijk C-O, C-B en C-O-C, die zich bonden bij respectievelijk 283,7 eV, 285 eV en 287 eV. Vanwege de lage samenstelling van C en een 1:1-stoichiometrie van B en N in de BGH-reeks, speculeerden we verder dat de structuur van BGH01 C en O-gedoteerd h-BN is. De voorgestelde structuur werd ondersteund door de vorming van CB-, B-O- en BN-O-bindingen binnen het hBN-domein, zoals blijkt uit de XPS-spectra (figuur 3). Het is dus redelijk om de structuur van de BGH-nanoschijven af te leiden die bij 800 ° C zijn bereid als C en O-gedoteerde hBN. Verder ondersteunden poeder-XRD en TEM met hoge resolutie de vorming van covalent gebonden B, C, N en O in een honingraatrooster met roosterafstand vergelijkbaar met die van turbostratisch boornitride. In dit manuscript zal de structuur van BGH01 worden aangeduid als BCNO-gedoteerde hBN.
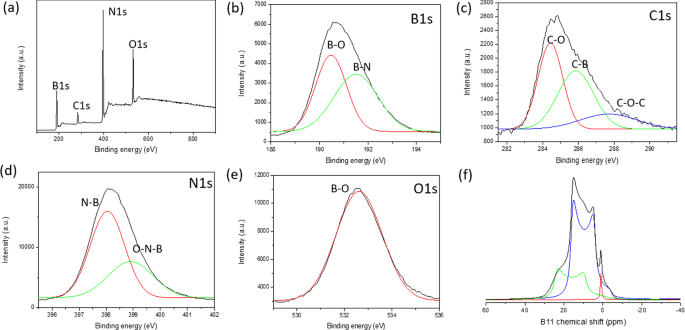
een XPS-enquêtespectra van BGH01. Kernniveauspectra van b B1s , c C1s , d N1s , en e O1s . Elke gedeconvolueerde piek is uitgerust met Gauss-functies. v De 11 B vastestof MAS NMR-spectra van BGH01. ( 11 B solid-state MAS NMR van BGH01-LT wordt getoond in aanvullend bestand 1:figuur S10)
We onderzochten ook het effect van calcineringstemperaturen en -tijden op de structuur-fotokatalytische activiteit van BCNO-nanostructuren, terwijl we de andere syntheseparameters constant hielden. Bij het verhogen van de reactietemperatuur (van 600°C tot 800°C) en het verhogen van de reactietijden van 30 minuten tot 12 uur, was er een algehele toename van BN- en B-O-binding (aanvullend bestand 1:figuur S6). Daarentegen neemt BC-binding af met toenemende reactietemperatuur en -tijd, wat de vorming van energetisch stabiele hexagonale B-N- en B-O-bindingen impliceerde terwijl de metastabiele BC-bindingen werden opgeofferd [48]. Zoals verwacht, neemt de B-C-bindingssamenstelling toe bij het verhogen van de verhouding van de hexamethyleentetramine-precursor (als de C-bron), terwijl de andere parameters constant worden gehouden [49]. Een meer gedetailleerde trend van de chemische bindingsevolutie van BGH bereid bij verschillende calcineringstemperaturen, tijden en voorloperverhoudingen wordt gegeven in de ESI (aanvullend bestand 1:figuur S6 en S7).
1 De 11 B solid-state MAS NMR werd gebruikt om elke B-gerelateerde bindingssamenstelling in BGH en BMH kwantitatief te analyseren. Hierin presenteren we de eerste gedetailleerde structurele karakterisering van BCNO op moleculair niveau met behulp van solid-state MAS 13 C en 11 B NMR om specifieke C- en B-gerelateerde bindingen te verzamelen. Hoewel hBN-nanomaterialen en hun defect-geïnduceerde elektronische eigenschappen aanzienlijke belangstelling hebben gewekt, zijn de moleculaire structuur van hBN-randen en defectenstructuur grotendeels onbekend [50]. Het gebrek aan structurele karakterisering van boorgerelateerde nanomaterialen is te wijten aan de moeilijkheid bij het analyseren van de vaste stof 11 B NMR-spectrum omdat 11 B is een half geheel getal quadrupoolkern (I = 3/2) [51, 52]. Halfgeleider 11 B NMR is ook een uitdaging om te interpreteren vanwege de tweede-orde quadrupoolkoppeling die resulteert in de vervorming van het signaal, die slechts gedeeltelijk kan worden gemiddeld via MAS NMR [53]. Verder is het bereik van de chemische verschuiving voor 11 B NMR is relatief smal, waardoor de piektoewijzing van de brede, overlappende en vervormde pieken van verschillende boorsoorten buitengewoon uitdagend is [54]. In dit onderzoek hebben we 11 . uitgevoerd B vaste stof NMR geregistreerd bij 9,4 T, en het spectrum werd gedeconvolueerd met behulp van topspin solid-line gevormde analyse (SOLA). Door de CP-MAS 11 . te volgen B NMR-experiment gerapporteerd in de literatuur, hebben we zinvolle B-gerelateerde chemische bindingsinformatie verkregen door rekening te houden met de quadrupolaire koppelingsconstante (C Q ) en de elektrische veldgradiënt (EFG) tensorasymmetrie (η Q ) [51, 52, 55]. Afbeelding 3f toont de solid-state 11 B spin-echo NMR-spectra van BGH met drie hoofdpieken en δ iso gecentreerd op respectievelijk 28,3 ppm, 20 ppm en 1,2 ppm. Gebaseerd op literatuuronderzoek naar CP-MAS 11 B NMR van boornitride en hun verwante structuren, de piek met een δ iso van 28,3 ppm (groene tracering) en een C Q van 2,85 MHz kwam overeen met de trigonaal-planaire BN2 (OH) soorten met een enkele hydroxylgroep [53,54,55]. Toen de hydroxylgroep of het zuurstofoverbruggende atoom het stikstofatoom rond de trigonaal-vlakke boorplaatsen verving, ontstond er een nieuwe B-soort met een δ iso van 20 ppm (blue trace fit) verscheen. Dit lagere chemische verschuivingssignaal werd hoogstwaarschijnlijk toegeschreven aan een andere trigonale boorplaats met twee hydroxylgroepen of één hydroxylgroep en één overbruggend zuurstofatoom (BN(OH)2 of BNO(OH)-plaatsen). De scherpe piek bij δ iso van 1,2 ppm (red trace fit) komt overeen met een tetraëdrische B-site met vier coördinaten, waarschijnlijk gecoördineerd door stikstof en meerdere hydroxylgroepen of een overbruggend zuurstofatoom (52-54) of koolstofgerelateerde CB-binding [46, 52]. De CP-MAS 11 B NMR-analyse van BGH01 onthulde verschillende B-N-, O-B- en BC-bindingen die evenredig zijn met de XPS- en XRD-analyses. Bovendien, solid-state 11 B NMR onthulde ook dat het meeste boor gebonden was met stikstof en met een of meer hydroxylgroepen als de BNx (OH)3−x soorten. Deze gehydroxyleerde soorten werden gespeculeerd om colloïdale stabiliteit in de waterige oplossing te bieden door waterstofbinding en elektrostatische stabilisatie. We zagen ook een afname in de tetracoördinaat B-soorten zoals BNx (OH)4−x of BNx (O)(OH)3−x (waarin O de overbruggende zuurstof is) en de boroxolringen [54, 56] bij toenemende reactietemperatuur. Tegelijkertijd zijn verschillende driecoördinaat BNx (OH)3−x soorten ontstonden toen de gloeitemperatuur steeg van 600°C naar 800°C (Aanvullend bestand 1:Figuur S8 en S10). Dit resultaat impliceerde dat bij hoge temperaturen de boroxolring reageerde met ammoniak om verschillende gehydroxyleerde BNx te vormen (OH)3−x of BNx (O)(OH)2−x soort [55] (Aanvullend bestand 1:Fig. S10).
Structurele analyse van BMH
Gebaseerd op onze grondige structurele analyse via XPS en FTIR en 11 B, en 13 C vaste stof MAS NMR, de structuur van BMH werd voorgesteld als BCNO-gedoteerde grafeenoxiden. Volgens de XPS-oppervlakte-elementanalyse bestond BMH voor 75% uit koolstofsoorten en slechts 5% uit boorgerelateerde soorten (aanvullend bestand 1:tabel S3). De gedeconvolueerde B1s spectra toonden BCN- en BN-binding bij respectievelijk 191,7 en 192,4 eV bindingsenergieën (Fig. 4). De C1s soorten vertoonden twee verschillende foto-emissiesignalen, die overeenkwamen met C-C-binding (sp 2 en sp 3 C-C-binding) met bindingsenergie van ongeveer 285.0 eV, en een zwakkere component kwam voort uit de BC-N-binding die verscheen bij 285,6 eV [57]. De andere relatief kleine signalen bij 288,0 eV en 288,7 eV waren te wijten aan C-N3 en C = O binding, respectievelijk [46, 57, 58]. Er werd gespeculeerd dat het geoxygeneerde C-atoom (C = O) zich zou vormen aan de randen van de grafeenoxidedomeinen, en de C-N3 binding was de karakteristieke piek voor CN. De N1s XPS-spectra, gecentreerd op 399,0 eV bindingsenergie, kunnen worden uitgerust met twee Gauss-curven bestaande uit CNB bij 399,2 eV binding en C = N binding bij 400,0 eV [46, 57, 59, 60]. Op basis van XPS-analyse omvatte de BMH-serie slechts ongeveer 10-25% O-gerelateerde binding vergeleken met ongeveer 35% O-gerelateerde binding in de BGH-serie. De O1s spectra gecentreerd op 531,2 eV bindingsenergie kunnen worden gedeconvolueerd in C = O- en CO-binding, wat kan worden toegeschreven aan het grafeenoxiden-domein. De 13 C solid-state CP-MAS NMR toonde verder de aanwezigheid van grafitische C = C-binding bij 40,2 ppm aan, wat overeenkomt met de XRD- en XPS-resultaten [38] (Fig. 5). In elk van de BMH-series die in dit artikel worden gerapporteerd, zijn de 13 C NMR toonde twee equimolaire verhoudingen van koolstofsoorten bij 160 en 154 ppm, overeenkomend met C α en C β , die meestal wordt aangetroffen in grafiet-koolstofnitridestructuren [61] (Fig. 5 en Aanvullend bestand 1:Tabel S4). Compared to the bulk CN synthesized according to the literature procedure [34], the chemical shift of the signature resonance for C α and C β peak appeared at 164 and 156 ppm, respectively (Fig. S13). Boron doping into the CN heptazine structure could have contributed to these slight chemical shift differences between the BMH series and the bulk CN (Additional file 1:Fig. S13).
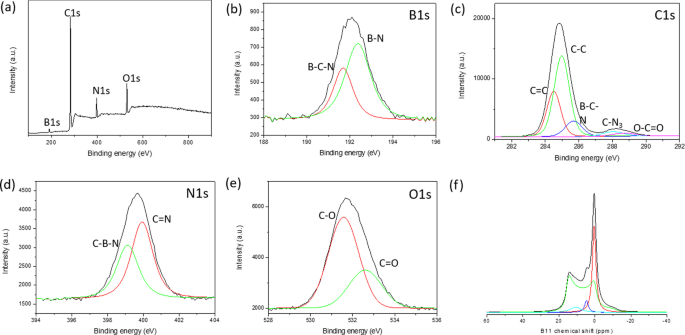
a Survey XPS spectra of BMH01 and 11 B NMR spectra. Core level spectra of b B1s , c C1s , d N1s , and e O1s . Each core spectra were fitted with a black trace, while the red and green traces under the peak were deconvoluted using a Gaussian function. f 11 B solid-state MAS NMR were deconvoluted using SOLA analysis to tricoordinate and tetracoordinate B-sites. The XPS and 11 B solid-state MAS NMR of other BMH series compounds can be found in Additional file 1:Figure S7, S11, and S12)
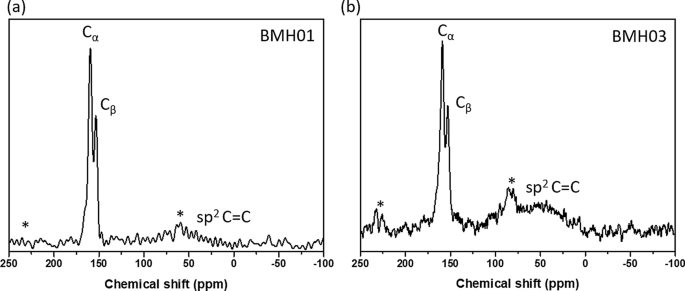
Solid-state 13 C MAS NMR of a BMH01 and b BMH03
Due to the abundant evidence of the presence of carbon nitride (CN) in the BMH series based on solid-state NMR spectroscopy (Fig. 5), we considered three possibilities of interactions between BCNO-doped graphene oxide and CN in BMH, namely:(i) bulk phase separation, (ii) disordered 2D network, and (iii) layered intercalation [62]. To eliminate the possibility of bulk phase separation, we examined the XRD pattern of CN nitride andBMH as well as a physical mixture of both in a 1:1 mass ratio. We found that the XRD patterns of the mixtures showed only the diffraction pattern of the bulk CN with a slight reduction in the crystallinity compared to the pristine CN diffraction [63] (Additional file 1:Fig S4). Since the XRD pattern of BMH did not possess any diffraction peaks that corresponded to CN, this experimental result confirmed that CN did not form as a bulk-separated domain during the synthesis of BMH (Additional file 1:Fig. S4). We also considered the formation of a disordered 2D network, in which CN and the doped-graphene oxides are bonded on the same 2D plane [62]. Based on a literature report on a CN/graphene oxide 2D matrix, the XRD pattern of a disordered 2D network showed characteristic peaks for both species with a slight peak broadening and a slight peak shifting [64]. However, the XRD pattern of BMH (Fig. 2) did not contain any signature diffractions of CN. A previous study also showed that characteristic peaks of graphene oxide disappeared in a graphitic CN/amorphous CN/graphene oxide composite due to the layer-by-layer interactions [65]. Therefore, it is reasonable to propose that CN is intercalated between the doped-graphene oxide layers. The FTIR spectrum of the selected BGH and BMH series is shown in Additional file 1:Fig. S5.
Boron-related bonding within the BMH series was investigated using 11 B solid-state MAS NMR at 9.4T, and the broad NMR spectrum was deconvoluted using the SOLA analysis showing the presence of both tricoordinate and tetracoordinate boron site. The SOLA analysis yielded four line fittings under the broad 11 B NMR spectrum, which could be assigned as trigonal planar BN2 (OH) or BN2 O at a δiso of 19.8 ppm and a CQ of 2.85 MHz (green fitting). The bay and corner B sites as in B-doped CN appeared at a δ iso of 5 ppm and δ iso of 11 ppm, respectively [61]. These assignments are also commensurate with the formation of CN based on the 13 C NMR and XPS analyses. Compared to the BGH series, the relative composition of the tetracoordinate B(IV) site of BMH was much higher (ca. 55% in the BMH series vs. 3% in the BGH series). However, the tetracoordinate B(IV) species in BMH appeared at a lower chemical shift than those found in BGH (Fig. 3f) and was therefore presumed to be the BN2 (OC)2−x (OH)x species [46]. Notably, the h-BN domain was absent from the BMH series prepared via thermal annealing at a lower temperature (600 °C). However, upon increasing the thermal annealing temperature from 600 °C to 800 °C, the structures of BMH01HT-30 min and BMH01HT-12 h showed a high composition of tetracoordinate BN2 (OH)2 species and the tricoordinate BN3 bonding (Additional file 1:Fig. S11 and S12). The presence of a high composition of tetracoordinate BN2 (OH)2 and BN3 bonding was shared among all the inactive BCNO investigated in this study. Moreover, although BMH01HT-12 h possessed an identical surface elemental composition to that of BGH01, the solid-state 11 B NMR revealed that both compounds possessed significant structural differences, which explained for their differences in photocatalytic activity (Additional file 1:Table S3 and Fig. S12).
In light of the moderate photocatalytic activity of BMH01 and BGH01 (Fig. 7), further synthesis optimization was performed to expand their light absorption spectrum into the visible light region. Previous literature showed that increasing the composition of the hexamethylenetetramine precursor (as a carbon source) could modulate the bandgap and photoluminescence properties of BCNO. Based on these reports, BMH and BGH compounds with a higher ratio of hexamethylenetetramine were prepared accordingly while keeping the other parameters constant. The optimized BCNO with a higher carbon content is denoted as BMH03 and BGH03, in which the molar ratio of carbon source was increased from 0.1 to 0.3. The higher ratio of hexamethylenetetramine precursor yielded BMH03 with a higher composition of the graphitic domain as in sp 2 C = C, and a small peak emerged which corresponded to BCN bonding at 191 eV binding energy (Additional file 1:Fig. S7). The increase in the graphitic sp 2 C = C domain upon increasing the concentration of hexamethylenetetramine is consistent with the role of hexamethylenetetramine as both a C and N source in the synthesis of N-doped graphite [66]. The increased sp 2 C = C graphite bonding in BMH03 was further confirmed via 13 C CP-MAS NMR with the emergence of a more prominent peak centered at 40.2 ppm, which is the signature of graphitic C = C(H) bonding (Additional file 1:Table S4).
Optical Properties
The optical properties of BMH and BGH were investigated using UV–visible absorption and photoluminescence spectroscopy, as shown in Fig. 6. Since BGH and BMH series possessed distinctively different structures, and the optical properties of nanomaterials are highly correlated with their structures, the origin of absorption and luminescence for both series were also found to be different. In this study, BGH01 quasi-spherical nanoparticles showed a featureless UV–vis spectrum. (Fig. 6a, red trace). As the ratio of hexamethylenetetramine increased, the intensity of absorbance peaked at 237 nm for BGH03 and increased with the emergence of an additional broad absorption peak centered around 330 nm (Fig. 6a, blue trace). The origin of the optical properties of BCNO is controversial due to the lack of structural analysis of BCNO nanomaterials. The most widely cited origin of photoluminescence of BCNO is attributed to the formation of B, C, N, and O self-interstitial sites, substitutional impurities, and native point defects within hBN or the graphene matrix [50, 67, 68]. Other possible photoluminescence mechanisms in the BCNO system include the electronic transition from the nitrogen-vacancy (VN ) levels to the carbon impurity levels, the electronic transition between the closed-shell BO − and BO 2− anions, and intrinsic state emission and defect state emission (surface energy traps). The featureless UV absorbance of BGH01 can be attributed to the electronic transition between the valence band (VB) and conduction band (CB) of boron nitride with a bandgap energy of ca. 5.9 eV. The lower energy absorption in BGH03 was a result of the mid-gap absorption from the valence band to the nitrogen-vacancy (VN ) level located approximately 0.7–1.0 eV below the conduction band of hBN.(62) The absorption peak at ca. 330 nm could be attributed to the presence of C-related impurities level located ca. 2–4 eV below the conduction band of hBN [69,70,71]. Under 365 nm excitation, BGH01 produced a broad emission with three bumps located at 412 nm, 445 nm, and 489 nm, respectively. Based on the BGH structural analysis, in which the dominant structure was composed of BN and BO-related bonding, the photoluminescence of the BGH series could be most likely originated from the B-O luminescence centers [24, 47, 72]. The yellow-green emission at 445 and 489 nm could be induced by the transition from the VN level to the carbon-related and oxygen defect levels (2–4 eV) below the conduction band of h-BN [69,70,71]. As the C composition increased in BGH03, the emission wavelength was further red-shifted to 506 nm, consistent with literature reports [73, 74]. The stacked photoluminescence (PL), UV absorbance, and Tauc plot for different BGH series prepared in this study are presented in Additional file 1:Fig. S14.
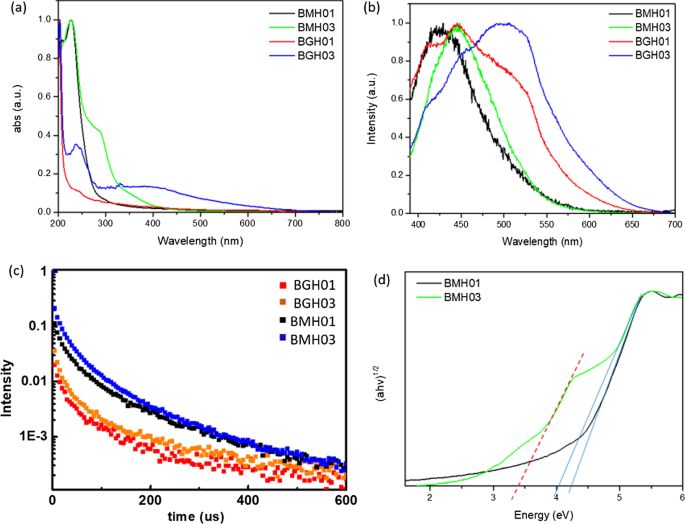
a Overlay UV absorbance spectra of BMH01, BMH03, BGH01, and BGH03. b Stacked photoluminescence spectra of BMH01, BMH03, BGH01, and BGH03 upon excitation at 365 nm c stacked photoluminescence lifetime decay spectrum of BMH01, BMH03, BGH01, and BGH03 monitored at a predetermined λ max for each sample. Samples were excited with 337 nm laser pulses at 298 K, d Overlay Tauc plot (αhv) 1/2 vs. hv, for BMH01 (black trace) and BMH03 (green trace). The optical band gap is represented by thesolid line, while the interband state for BMH03 is represented by the red dashed line
Based on XRD and various spectroscopic analyses, the structure of BMH was deduced to be dominated by BCNO-doped graphene oxides (Fig. 4, Additional file 1:Fig. S7, and Fig. S12, and Table S4). Thus, the optical properties of the BMH series are hypothesized to be more closely related to the carbon-quantum dot (CD) [75, 76] and doped-graphene oxides systems [44, 77,78,79]. Based on the origin of photoluminescence of the CD and graphene oxides, the optical properties of BMH prepared in this study can be attributed to the intrinsic state emission [76, 80, 81], electron–hole recombination [82, 83], and defective state emission [84]. Intrinsic emission of BMH is speculated to have originated from isolated sp 2 luminescence centers embedded within the sp 3 matrix of the carbonaceous film. The sp 3 matrix of graphene oxides is composed of C–OH, C–O–C, and C = O edge sites, whose energy levels lie between the energy levels of π–π* states of the sp 2 C = C domain, thus giving rise to multiple absorption bands. Both BMH01 and BMH03 possessed a strong UV absorbance band at ca. 240 nm, corresponding to the π to π* transition of C = C within the graphene oxides domain. With the increasing ratio of hexamethylenetetramine in the BMH03 sample, an additional bump at 288 nm emerged, which can be ascribed to the n-π* transition of the C = O and C = N bonds of the oxidized graphitic region [77, 85, 86]. The latter absorbance band at ca. 288 nm was induced by oxygen, nitrogen, and boron defect sites, creating new radiative recombination sites [82, 87,88,89]. Upon photoexcitation at 365 nm, BMH01 and BMH03 exhibited a maximum emission wavelengths at 429 and 447 nm, respectively. The BMH03 sample showed a slight red-shift emission, unlike BMH01 due to the greater extent of graphitization [77, 84] (Additional file 1:Figs. 5 and 6). Both BMH samples revealed a broad and much lower energy emission wavelengths than BGH samples due to lower energy emissive centers arising from O, N, and B defects and surface states. According to the electron–hole recombination mechanism, these photoexcited electrons from each defect state recombine with their corresponding holes in the HOMO, thus yielding a broad photoluminescence emission [90] (Fig. 6b). Interestingly, only BMH01 exhibited a pronounced excitation-dependent photoluminescence as observed in other BCNO [72] and carbon quantum dot systems [76]. The presence of O, and N impurities embedded within the graphene oxides matrix was shown to create a large number of surface emissive traps that corresponded to a diverse energy levels within the bandgap, thus yielding an excitation dependent fluorescence spectra in BMH01 (Additional file 1:Fig. S15). In contrast, the lack of an excitation dependent emission in BMH03 could be explained by the formation of a greater extent of graphitization (C = C) with a concurrent reduction in the surface states population (eg.:C = O) [44].
The bandgap values of BMH and BGH prepared in this study were estimated from Tauc's formulation:(αhν) 2 − hν, where α is the absorbance (Fig. 6d). The bandgap was estimated by extrapolating the photon energy intercept at (αhν) 2 = 0. For the BMH series, the presence of multiple energy levels within the optical bandgap may have originated from the electronic transition from various π-π* (C = C bonds) and n-π* of C = O or other surface groups [76, 82, 87]. As for the BGH series, carbon substituted on boron sites (CB ), nitrogen-vacancy sites (VN ), and interstitial carbon defect levels gave rise to the emergence of interband states between the bandgap of hBN. The presence of multiple energy levels was supported by the photoluminescence spectra of lower energy radiative recombinations [24, 47, 66] (Fig. 6b and Table 2). The BGH03 sample exhibited two large bandgaps at 5.7 eV and 3.8 eV, corresponding to the bandgap of hBN and the transition from the valence band to VN levels, respectively [50, 74] (Additional file 1:Fig. S14).
Long-lived charge carriers that can persist into the microseconds and milliseconds timescales in semiconductor photoelectrodes such as CN photocatalysts, have been proposed as an important parameter in enhancing photocatalytic activity by reducing charge recombinations [91,92,93,94,95]. Time-resolved photoluminescence (TRPL) experiments were conducted to gain insight into the recombination processes of the photogenerated charge carriers of BCNO (Fig. 6c). The µs-PL decay kinetics could be fitted with three exponential decays according to the following equation.
$$I\left( t \right) =I_{1} \exp ( - t/\tau_{1} ) + I_{2} \exp ( - t/\tau_{2} ) + I_{3} \exp \left( {t/\tau_{3} } \right)$$The multiple exponential decays imply that BCNO undergoes complex recombination from both intrinsic and defect states of BCNO [32, 72]. In the equation, I 1 through I 3 are constants with values of emission intensity measured at t =0, andτ 1 through τ 3 are the lifetimes of three channels responsible for the decay, respectively. Through multiexponential fitting of the entire decay curves for the BMH and BGH series, the average lifetimes were calculated to be 1.58, 2.10, 5.18, and 8.14 µs for BGH01, BGH03, BMH01, and BMH03, respectively. The values of I and τ in Eq. 1 for the BMH and BGH series are reported in Additional file 1:Table S5. The persistent lifetime of the charge carrier in the BCNO system has been attributed to the presence of shallow traps composed of nitrogen-vacancy (VN ) stabilized by carbon impurities, which were located ca. 0.7 eV-1.0 eV below the conduction band of h-BN [72, 74]. Shallow traps in CN photocatalysts have been attributed to charge separation states with long life-times due to chemical defects [95]. According to works related to prolonged photoluminescence in CN and other nanostructured photoelectrodes [91, 92], the microseconds lifetimes of BCNO-doped graphene oxides and BCNO-doped hBN are associated with the enhanced charged separation within the BCNO domain. The ultralong lifetimes are speculated to be a critical factor in facilitating heterogeneous photocatalysis [91].
Photocatalytic Dye Degradation
As a proof of concept demonstration, the photocatalytic performance of BMH and BGH was evaluated by the photodegradation of methylene blue (MB) under UV–visible light irradiation. Details of the experimental procedure and analysis of the photocatalytic dye degradation are available in the ESI. Figure 7 shows the percent degradation (C /C 0 × 100%) for BMH03 (red line), BMH01 (blue line), BGH01 (green line), BGH03 (orange line), and CN (purple line), where C is the concentration of MB at a time, t and C 0 is the initial concentration MB after the dark equilibrium. According to the Langmuir–Hinshelwood model, ln(C /C 0 ) = kt , where k is the rate constant, the dye degradation rate constant values were calculated to be 2.31 × 10 −3 min −1 for BMH03, 1.52 × 10 −3 min −1 for BGH01, 1.48 × 10 −3 min −1 for BMH01 and 9.38 × 10 −4 min −1 for BGH03. Compared to the state-of-the-art metal-free photocatalyst, BMH03 exhibited a 25% improvement in the photocatalytic dye degradation rates (Fig. 7). This proof-of-concept demonstration warrants a more in-depth investigation of the structure–property relationship of this new metal-free, boron-based photocatalyst.
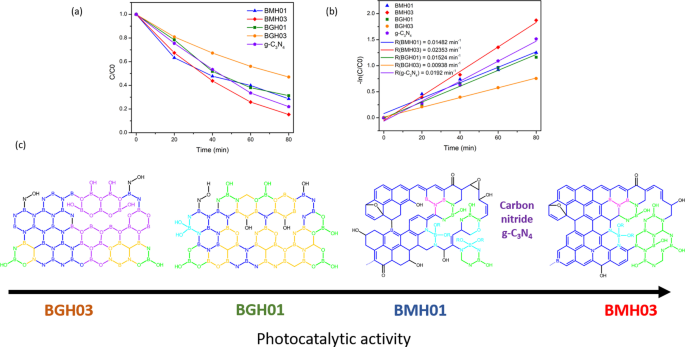
UV–visible light-induced photocatalytic MB degradation using BGH and BMH. a Photodegradation of MB under UV–visible light (plot of C /C 0 ) and b pseudo-first-order rate reaction kinetics for MB dye using BGH, BMH, and CN as a photocatalyst. c The proposed structures for BGH01, BGH03, BMH01, and BMH03 and their corresponding activities toward photodegradation of MB dye. Each domain is color-coded, i.e., BCNO (orange), h-BN and graphene oxides (blue), tricoordinate boron BN2 (OH) and BO2 (OH) (green), boroxol ring (purple), tetracoordinate B sites (aqua blue), and functional groups or dangling bonds (black).
Based on the detailed structural analysis and the proposed structure in Fig. 7c, the highest photocatalytic activity of BMH03 consisted of BCNO-doped graphene oxides [1, 65]. This ternary metal-free photocatalyst is reported to enhance the photocatalytic performances by increasing the charge separation and migration to the reaction site [1]. Additionally, the incorporation of boron into graphene-based materials [11, 20], CN [21, 96], and carbon nanotubes [97] has also exhibited enhanced performance compared to their pristine material without a dopant due to multiple synergistic effects. The large differences in electronegativity between boron, carbon, and nitrogen (2.04 vs. 2.55 vs. 3.04, respectively) yielded a strongly polarized bonding towards C and N atoms. As a result, a local positive charge was formed on boron that turned boron into a strong acidic defect site for preferential adsorption sites of pollutants [98], O2 [97], HOO
−
and OH
−
[99, 100]. Therefore, electron-rich pollutants such as MB (used as a model reaction) were speculated to preferentially adsorbed onto the electropositive B sites of the BMH photocatalyst. The photocatalytic degradation of MB on BCNO was proposed to undergo an indirect dye degradation mechanism [101]. In the indirect photodegradation mechanism, the photogenerated holes on the surface of BCNO produced highly oxidative hydroxyl radicals and attack the bond of MB. Meanwhile, photogenerated electrons from the conduction band of BCNO formed highly reducing superoxide radical anion O2
−
species that could directly attack MB. Figure 8 illustrates the proposed mechanism of BCNO in photocatalyzing the degradation of MB
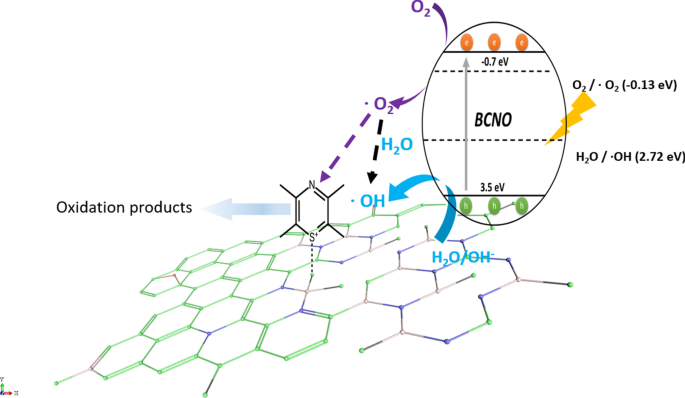
Schematic illustration of the proposed mechanism of BCNO in catalyzing the degradation of MB upon light irradiation. The positively charged MB was selectively absorbed on the N2 B-OH sites. The band structures of BMH03 were determined via UPS and Tauc plot (Fig. 6 and Additional file 1:Fig. S17). The redox potentials of O2 /O2 = − 0.13 eV vs ENHE and H2 O/OH = 2.72 eV vs. ENHE are given in black dashed lines as reference
According to the XPS and solid-state NMR analyses, BMH03 possessed a higher composition of graphitic sp 2 C = C with a simultaneous reduction in tetracoordinate B site compared to BMH01 (Additional file 1:Fig. S9 and Table S4) The high composition of tetracoordinate B-sites in BMH01 (33%) also translated to a reduction in the number of boron Lewis acid sites serving as the active catalytic center, which explains the trend of photocatalytic activities among the BMH series [13]. However, the BGH series comprised the C, and O-doped h-BN domain with various tri- and tetracoordinate boron sites. Interestingly, BGH01 annealed at a lower temperature (Table 1, BGH01LT) was found to be inactive but exhibited a high absorbing ability in removing dye from the solution (Additional file 1:Fig. S1 for TEM morphology; Fig. S16 for photodegradation MB UV–vis absorbance).
The previous report also concluded that BCNO was photocatalytically inactive towards dye degradation but exhibited an excellent absorbing ability in removing dye from the solution [102]. The inactive BGH01LT was comprised primarily of tetracoordinate B sites and boron oxides-related bonding. Based on the SOLA analysis and the literature , the tricoordinate boron site with a δ iso of 16.7 ppm was related to boroxol rings (B2 O3 ) [54, 56]. Our results are also supported by other works on the catalytic activation of peroxymonosulfate using amorphous boron [22]. However, the photocatalytic active BGH01, BMH01, and BMH03 possessed a high composition of BN(OH)2 and BN2 OH bonding sites. Our investigation suggested that the photocatalytic activity of BCNO is highly dependent on the local structure of the boron site. While the exact catalytic site and mechanism are yet to be explored, we proposed that BNx (OH)3−x served as one of the BGH and BMH series catalytic sites. For the BMH series, the formation of tetracoordinate B-O sites was detrimental in catalyzing dye degradation due to the reduction in the Lewis acid site. At the same time, the increasing composition of the sp 2 C = C graphitic domain enhanced the photocatalytic activities of the BGH and BMH series.
Conclusions
In summary, BCNO structures and their photocatalytic activities have been presented here and found to be highly dependent on the choice of precursor, precursor ratios, annealing temperatures, and times. In this study, two types of distinctly different BCNO nanostructures were prepared via low-temperature annealing (600 °C–800 °C):(1) BCNO-doped boron nitride and (2) BCNO-doped graphene oxides. Through systematic investigation of using two different nitrogen precursors, crystalline BCNO with a quasi-spherical shape was prepared at 800 °C for 12 hr using guanidine hydrochloride as the nitrogen source. This series of BCNO exhibited moderate photocatalytic activity through the emergence of BN2 (OH) or BN(OH)2 tricoordinate boron serving as the Lewis acidic site. However, BCNO prepared using melamine as the nitrogen source at 600 °C yielded multi-layered sheets with ill-defined shapes. These BCNO-doped graphene oxides layered structures exhibited the highest photocatalytic activity, surpassing the state-of-art metal-free photocatalyst, CN. For the melamine-derived BCNO layered structures, the presence of tricoordinate boron species as BN2 (OH) or BN(OH)2 and a higher composition of graphitic sp 2 C = C were speculated to play an important role in promoting their photocatalytic activity. This study demonstrates the potential of BCNO as a photocatalyst for energy conversion and environmental remediation applications. Further structural optimization on this new B-C-N–O photocatalyst system is expected to facilitate the development of a sustainable catalyst for applications including solar hydrogen fuel production, environmental remediation, electrocatalytic oxygen reduction reaction, and catalytic oxidative dehydrogenation reaction.
Availability of Data and Materials
All data are fully available without restriction.
Abbreviations
- BCNO:
-
Boron carbon oxynitride
- CN:
-
Carbon nitride
- hBN:
-
Hexagonal boron nitride
- TRPL:
-
Time-resolved photoluminescence
- MAS:
-
Magic angle spin
- CP:
-
Cross polarization
- SOLA:
-
Solid line shape analysis
- I :
-
Intensity
- ATR:
-
Attenuated total reflectance
Nanomaterialen
- Koolstofvezel
- Koolmonoxidedetector
- Carbonpapier
- Carbon M2
- Koolstof nanobuisjes verleggen de limiet van flexibele elektronica
- Cyclocarbon maken en in beeld brengen
- Ocean Carbon afgebeeld op de atoomschaal
- Koolstof nanobuisgaren, spierweefsel en transparante vellen
- Wat is koolstofvezel?
- Is koolstofvezel geleidend?
- Koolstofstaal versus roestvrij staal