Carbon Dots als nieuwe generatie materialen voor nanothermometer:Review
Abstract
Zeer gevoelige contactloze temperatuurmeting is essentieel voor het bestuderen van fundamentele chemische reacties, biologische processen en toepassingen in de medische diagnostiek. Op nanoschaal gebaseerde thermometers garanderen niet-invasieve sondes voor gevoelige en nauwkeurige temperatuurmeting met subcellulaire resolutie. Op fluorescentie gebaseerde temperatuursensoren hebben een grote capaciteit getoond omdat ze werken als "contactloze" modus en de dubbele functies bieden van cellulaire beeldvorming en het meten van de temperatuur op moleculair niveau. Vooruitgang in nanomaterialen en nanotechnologie heeft geleid tot de ontwikkeling van nieuwe sensoren, zoals nanothermometers (nieuwe temperatuurgevoelige materialen met een hoge ruimtelijke resolutie op nanoschaal). Dergelijke nanothermometers zijn ontwikkeld met behulp van verschillende platforms zoals fluorescerende eiwitten, organische verbindingen, metalen nanodeeltjes, met zeldzame aarde gedoteerde nanodeeltjes en halfgeleider kwantumstippen. Carbon dots (CD's) hebben belangstelling gewekt in veel onderzoeksgebieden vanwege uitstekende eigenschappen zoals sterke fluorescentie, fotobleking-resistentie, chemische stabiliteit, goedkope voorlopers, lage toxiciteit en biocompatibiliteit. Recente rapporten toonden het thermische detectiegedrag van sommige cd's aan, waardoor ze een alternatief zijn voor andere op nanomaterialen gebaseerde thermometers. Dit soort op luminescentie gebaseerde thermometer is veelbelovend voor temperatuurmeting in nanoholtes en thermische mapping om een beter begrip van biologische processen te krijgen. Met cd's die nog in de kinderschoenen staan als materiaal op nanoschaal voor thermische detectie, bieden we in deze review een uitgebreid begrip van deze nieuwe nanothermometer, functionaliseringsmethoden om de thermische gevoeligheid en resolutie te verbeteren, en het mechanisme van het thermische detectiegedrag.
Inleiding
Temperatuur is een fundamentele thermodynamische variabele die een opmerkelijke invloed heeft op de biologische en chemische systemen. Vanwege het brede scala aan toepassingen, bijna op alle gebieden van natuurwetenschappen, techniek, landbouw en medische wetenschappen, is nauwkeurige temperatuurbepaling van groot belang [1, 2]. In medische toepassingen wordt thermometrie gebruikt voor de vroege detectie van verschillende ziekten, zoals beroerte, kanker of ontstekingen, waarvan een van de eerste symptomen het optreden van plaatselijke temperatuurafwijkingen is.
In de geschiedenis was de vroegste schatting van temperatuur mogelijk gebaseerd op sensatie of observatie. In de oudheid, 200-10 v. Chr., zijn pneumatische experimenten (uitzetting van lucht door warmte) de oudste erkende referenties van apparaten die worden gebruikt voor kwantitatieve metingen van warmte. Te midden van de meest primitieve geschriften die betrekking hebben op door warmte uitgezette lucht worden de werken toegeschreven aan de werken van Philo van Byzantium en Hero (of Heron) van Alexandrië over pneumatische experimenten [3]. Later, tussen de jaren 1592 en 1603, vond Galileo Galilei een thermoscoop uit door experimenten uit te voeren met de uitzetting van lucht door warmte via de bouw van een eenvoudig apparaat met behulp van een buis die lucht omvat die boven een waterkolom is opgesloten. Na Galileo is het Italiaanse Santorio als eerste geaccrediteerd voor het integreren van dit eenvoudige apparaat in medische onderzoeken van koorts. De eerste volledig afgesloten vloeistof-in-glas thermometer, met alcohol gevulde glazen buis, werd in het jaar 1641 door Ferdinand II geassembleerd. Hij was in staat om de temperatuur te meten zonder hulp van barometrische druk, in tegenstelling tot de open thermoscoop van Galileo en Santorio. Fahrenheit's praktische werk in thermometrie ontstond in 1706; hij begon met alcohol maar werd later legendarisch voor zijn kwikthermometers. Erkenning voor de centesimale temperatuurschaal is gegeven aan Anders Celsius, die in 1742 een schaal projecteerde met nul bij de temperatuur van kokend water en 100 bij de temperatuur van bevriezend water. Elektronische experimenten uitgevoerd in de negentiende eeuw toen Thomas Johann Seebeck het concept thermo-elektriciteit overwoog. In een opeenvolging van experimenten uitgevoerd tussen de jaren 1820 en 1823, verifieerde hij de elektrische potentiaal in de verbindingspunten van twee verschillende metalen wanneer er een warmteverschil is tussen de verbindingen. Dit werd later het Seebeck-effect genoemd en het dient als de oorsprong van het thermokoppel dat wordt beschouwd als de meest nauwkeurige meting van temperatuur [3,4,5,6,7]. De schematische tijdlijn van de thermometer wordt getoond in Fig. 1.
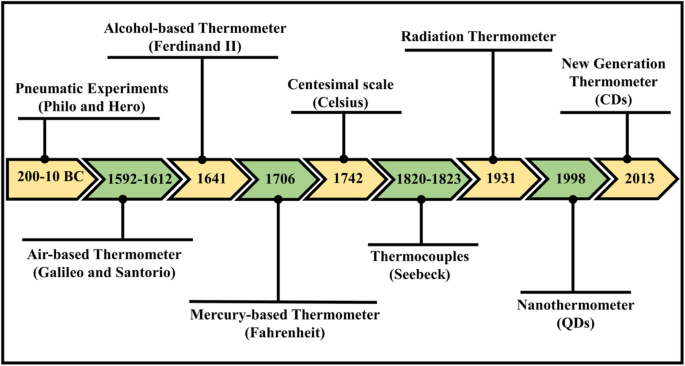
Tijdlijnschema voor de evolutie van thermometers
Conventionele thermometers kunnen worden onderverdeeld in:
- 1.
Met vloeistof gevulde glazen thermometers gebaseerd op de thermische uitzetting van materialen
- 2.
Thermokoppels gebaseerd op het Seebeck-effect
- 3.
Optische sensoren [8]
Bovendien kunnen ze worden geclassificeerd als contact- of contactloze thermometers. Contactmodus, inclusief klassiek met vloeistof gevuld glas, thermokoppels, thermistoren en weerstandstemperatuurdetectoren (RTD's), vereisen allemaal elektrische bekabeling en een directe aanraking tussen de thermometer en het substraat. Deze modus is niet geschikt voor toepassingen waarin elektromagnetische ruis sterk is, vonken gevaarlijk kunnen zijn, de omgeving destructief is of onderdelen snel in beweging zijn. Bovendien zijn traditionele thermometers niet in staat om te meten wanneer de ruimtelijke resolutie daalt tot de submicronschaal, bijvoorbeeld bij intracellulaire temperatuurvariaties en het in kaart brengen van de temperatuur van microcircuits en microfluïdica [9]. Op dezelfde manier vereisen technische toepassingen geavanceerde thermogevoelige strategieën voor miniatuurregio's en moeilijke omgevingen [10]. Voor domeinen op nanoschaal moet men dus nadenken over andere benaderingen en materialen.
Nieuwe contactloze thermometrie kan de bovengenoemde problemen overwinnen. Optische thermosensoren (moleculaire thermometers) zijn bijvoorbeeld een meer recente generatie analytische instrumenten die bestaat uit moleculaire klassen die de meting van uitgestraald licht gebruiken om de temperatuur te extraheren [11, 12]. Fluorescerende temperatuurgevoelige sondes bieden een veelbelovend gebied voor thermometrie in nanosysteemtoepassingen. De temperatuurinformatie kan worden geëxtraheerd op basis van hun fluorescentie-intensiteit, bandvorm, Stokes-verschuiving, of de levensduur van het verval kan de temperatuur relateren als deze correct is gekalibreerd [13, 14].
Moleculaire thermometers hebben een groot potentieel bij het diagnosticeren van zieke of kankerverwekkende cellen, die verschillende fysiologische temperaturen hebben dan gewone cellen. In de medische toepassingen variëren de mogelijkheden van temperatuurgeïnduceerde controle van genexpressie [15] en celmetabolisme [16] tot celselectieve penetratie en behandeling van ziekten [17] en verbetering van de warmteafvoer van geïntegreerde warmtebronnen [18] . Onlangs zijn nanomaterialen zoals halfgeleiders [19], polymere [10] en metalen nanodeeltjes [20] gebruikt als thermische sensor (nanothermometer) die een submicron thermische resolutie vertoonden.
Thermometers die sub-graden van temperatuur kunnen oplossen over een breed scala aan temperaturen en die ook kunnen worden geïntegreerd in levende systemen, zouden een invloedrijk nieuw hulpmiddel kunnen zijn op talloze gebieden van biologisch, fysisch en chemisch onderzoek. Daarom richten we ons in deze review op een "nieuwe generatie" of een "nieuwe klasse" nanothermometers die is gebaseerd op koolstofnanomaterialen (koolstofhoudende materialen). Voor zover ons bekend, is er geen gerapporteerd recensieartikel over koolstofstippen als nanothermometer. Onlangs vertoonden koolstofdots (koolstofkwantumdots, grafeenkwantumdots) samen met hun unieke eigenschappen gevoelige thermische eigenschappen, waardoor ze uitstekende kandidaten zijn voor thermometrisch gedrag op nanoschaal. Hier worden definities, voordelen en mechanismen van het thermische detectiegedrag van koolstofstippen besproken. Ten slotte worden toekomstperspectieven van deze nieuwe klasse van thermomaterialen gepresenteerd.
Nanothermometrie
Wat wordt bedoeld met "nano-thermometrie" is het gebruik van thermogevoelige materialen op nanoschaal om temperatuurinformatie te geven over de lokale omgeving van het nano- of microschaalgebied [21, 22]. Op nanodeeltjes gebaseerde thermische sondes hebben een grote capaciteit in een breed spectrum van detectietoepassingen, en de laatste tijd zijn er talloze progressieve zogenaamde "nanothermometers" gerapporteerd. Bovendien is gemeld dat verschillende soorten conventionele nanomaterialen warmtegevoelige lichtgevende eigenschappen hebben, zoals polymeren [23, 24], nanokristallen [25], met zeldzame aarde gedoteerde nanodeeltjes [26, 27] en metalen nanodeeltjes [28].
Nanothermosensoren zijn dus niet-invasieve, contactloze, nauwkeurige thermometers die op nanoschaal werken met een zeer gevoelige resolutie [9]. Thermische detectie met behulp van nanomaterialen kan worden bereikt door hun optische eigenschappen te manipuleren. Fluorescentie-nanothermometrie kan worden ingedeeld in nogal wat klassen, afhankelijk van de precieze parameter waaruit de thermische meting wordt afgeleid, waarbij de intensiteit van het signaal, de vorm van de band, de fluorescentie-levensduur, de bandverschuiving, de polarisatie van de excitatiegolflengte en de spectrale verschuiving betrokken zijn. In het eerste geval verandert de fluorescentie met verschillende temperaturen en kan worden gedetecteerd als een absolute toename (of afname) van het signaal [9, 29,30,31].
Contactloze luminescentie-nanothermometrie, waarbij gebruik wordt gemaakt van luminescente nanomaterialen met temperatuurafhankelijke emissies, is vooral geschikt voor biologische toepassingen [32, 33]. Deze lichtgevende nanomaterialen omvatten fluorescerende polymeren [24], metalen nanodeeltjes [34], met zeldzame aarde gedoteerde nanodeeltjes [35] en nanodiamanten [36] die een thermogevoelige eigenschap hebben in het fysiologische bereik. Deze baanbrekende werken waren in staat om de gemiddelde temperatuur voor individuele cellen te leveren. Temperatuurafhankelijke luminescentieprobes op basis van organische kleurstoffen (bijv. Rhodamine 6G) en polymeren (bijv. poly(N) -isopropylacrylamide)) vertonen over het algemeen een slechte fotostabiliteit en uitgesproken kruisgevoeligheid voor zuurstof, wat ongewenst is voor levend-celwerk [8]. Bovendien is er een sterke pH-afhankelijkheid van de levensduur van de fluorofoor, waardoor het moeilijk te gebruiken is zonder de nauwkeurige regeling van de pH van de omgeving die wordt onderzocht [37].
Een andere klasse nanothermometers op basis van zowel zuivere als gedoteerde halfgeleider nanokristallen is gerapporteerd, met de meest prominente kandidaten zoals CdSe, ZnS, InP of PbSe [19, 38,39,40]. Halfgeleider kwantumdots (SQD's) zijn een kandidaat voor thermometers op nanoschaal, aangezien ze een hoge kwantumopbrengst hebben, een lange levensduur vóór fotobleken en voldoende biocompatibiliteit na de juiste oppervlaktemodificatie. Bovendien kunnen ze gemakkelijk worden geconjugeerd aan eiwitten en DNA voor detectie en beeldvorming [41]. De uitzonderlijke uitdaging waarmee dit type lichtgevende thermometers wordt geconfronteerd, is de bijbehorende herkenning van helderheid, fotostabiliteit, gevoeligheid en precisie bij T =20-40 °C bij het onderzoeken van subcellulaire micro-omgevingen. SQD's zijn uitgebreid beoordeeld op het gebied van synthese, fysisch-chemische eigenschappen, luminescentie en hun potentiële toepassingen. Hier vestigen we de aandacht van de lezer op deze talrijke opmerkelijke recensies [42,43,44,45,46]. Vergeleken met organische kleurstoffen vertonen SQD's superieure helderheid voor detectie, een breder excitatieprofiel voor multiplexen en betere fotostabiliteit voor langetermijnstudies. Bovendien zijn SQD's als temperatuursensoren bestand tegen pH en andere omgevingsvariaties die naar verwachting in een cel voorkomen [47].
In het algemeen veroorzaakt een temperatuurstijging bij SQD's als gevolg van een combinatie van verschillende processen een afname van de fluorescentie-intensiteit (quenching), wat gepaard gaat met een spectrale verschuiving. Aangenomen kan worden dat deze verschuiving lineair is in het biofysische bereik. De omvang van beide effecten (uitdoving van de luminescentie en spectrale verschuiving) hangt sterk af van het materiaal waaruit de QD's bestaan en van hun grootte [48].
Elke groep lichtgevende nanomaterialen heeft naast hun voordelen ook beperkingen in het gebruik. Zoals hierboven beschreven, hebben SQD's meer de voorkeur dan fluorescerende polymeren en organische kleurstoffen. SQD's zijn goed in termen van fotostabiliteit, kwantumefficiëntie en afstembare fluorescentie, maar QD's kunnen vanwege hun intrinsieke knipperen niet worden gebruikt om een enkel molecuul te traceren voor langetermijnmonitoring [49]. Bovendien is de belangrijkste valkuil van QD's hun toxiciteit, die te wijten is aan hun gehalte aan zware metalen, waaronder metalen zoals cadmium; dit beperkt hun biologische en ecologische toepassingen. Bovendien is de beschikbaarheid van voorloperelementen in de natuur relatief laag en daarom worden SQD's als duur beschouwd [50].
Koolstofstippen als nanothermometers
Om problemen op te lossen die voortkwamen uit niet-koolstofgebaseerde nanothermometers (zoals we in de vorige sectie hebben uitgelegd), zijn op koolstof gebaseerde nanomaterialen bereid en vertoonden ze unieke eigenschappen, zoals lage toxiciteit, gemakkelijke bereiding, goedkope voorloper, fotostabiliteit en biocompatibiliteit. Deze op koolstof gebaseerde nanomaterialen vertoonden gevoelige thermische detectie-eigenschappen. Bovendien is de verbetering van metaalvrije nanodeeltjes belangrijk en urgent vanwege het milieugevaar voor biologische toepassingen van dergelijke giftige materialen [51, 52]. Onder de familie van op koolstof gebaseerde nanomaterialen zijn fluorescerende nanodiamanten voor het eerst gerapporteerd als nanothermometers [53]. Fluorescerende nanodiamanten bezitten intrinsieke biocompatibiliteit als gevolg van hun chemisch robuuste en inerte oppervlak [54]. Andere nanodiamanten zijn onlangs gebruikt voor intracellulaire thermische detectie met een nauwkeurigheid tot onder de graad [55, 56]. De thermische gevoeligheid van deze nanodiamanten is gebaseerd op de zogenaamde stikstof-vacature kleurcentra, dit zijn puntdefecten die bestaan uit een stikstofatoom dat een roosterkoolstofatoom vervangt en een nabijgelegen lege roosterlocatie [48, 57]. Het stikstof-vacaturecentrum van fluorescerende nanodiamanten wordt uitgebreid bestudeerd en is goed gekarakteriseerd vanwege zijn fotofysica, evenals zijn gebruik in biologische toepassingen [58]. Het werkingsprincipe van op stikstof-leegstand gebaseerde thermometrie hangt af van de nauwkeurige meting van dit kleurcentrum, dat optisch kan worden gedetecteerd met een hoge ruimtelijke resolutie [30, 59]. Lage fluorescentie-efficiëntie en slechte beheersbaarheid belemmeren echter de toepassing van fluorescerende nanodiamanten enorm [36].
Een van de nieuwere klassen van op koolstof gebaseerde nanomaterialenfamilies zijn zeer lichtgevende koolstofpunten (CD's), die een uitzonderlijke heldere fotoluminescentie, fotochemische stabiliteit, oplosbaarheid in water, grote biocompatibiliteit en niet-toxiciteit hebben [60,61,62]. Cd's zijn nuldimensionale en bolvormige nanodeeltjes met een diameter van minder dan 10 nm [63, 64]. Er zijn verschillende benaderingen toegepast voor het maken van diverse soorten cd's, bijv. laserablatie [65], solvotherme [66], hydrothermische synthese [67], microgolf-ondersteunde [68], boogontlading [69], zure oxidatie [70], en meer chemische en fysische benaderingen [71, 72]. Bovendien vertonen cd's een wenselijk perspectief voor verschillende toepassingen, zoals biologische beeldvorming [73], chemische en biosensing [74,75,76,77], gerichte medicijnafgifte [78], farmaceutische analyse [79] en katalyse [80, 81]. Koolstofstippen zijn uitgebreid beoordeeld op het gebied van synthese, fysisch-chemische eigenschappen en hun potentiële toepassingen. Hier verwijzen we de lezer naar de talrijke goede recensies over carbon dots [72, 82,83,84,85,86,87,88].
De laatste jaren hebben fluorescerende cd's als temperatuursensoren veel aandacht getrokken van onderzoekers. In principe zijn er enkele vereisten nodig voor de effectieve temperatuurmeting, zoals koolstofnanodots moeten een aanzienlijke variatie vertonen in hun fotoluminescentie over het bereik van relevante temperaturen [89]. De fotostabiliteit, pH-stabiliteit en houdbaarheid zijn andere vereisten waarmee rekening moet worden gehouden voor praktische toepassing.
Cd's zijn veelbelovende alternatieven voor conventionele halfgeleider kwantumdots (SQD's). Vergeleken met QD's vertonen cd's veel uitstekende voordelen, zoals lage kosten, lage toxiciteit en hun unieke robuuste optische/chemische eigenschappen [90]. Bovendien vertonen cd's zeer weinig fotobleking. In vergelijking met andere fluorescerende grondstoffen worden cd's geproduceerd uit goedkope koolstofbronnen die overvloedig in de natuur voorkomen [91]. Verder zijn er verschillende eenvoudige methoden om de oppervlaktetoestand van cd's te wijzigen en te functionaliseren, waardoor de oplosbaarheid, stabiliteit, fysisch-chemische eigenschappen en kwantumopbrengsten van cd's kunnen worden aangepast aan hun experimentele vereisten [49, 92, 93].
In de literatuur is er een schaars aantal gerapporteerde artikelen van koolstofstippen met temperatuurafhankelijke fluorescentie en worden weergegeven in tabel 1.
Yu et al. [51] waren in 2012 de eersten die de temperatuurafhankelijke fluorescentie in koolstofnanodots onderzochten en vergeleken met halfgeleiders en op metaal gebaseerde nanodeeltjes. Ze waren afhankelijk van het meten van de temperatuurafhankelijke levensduur van fotoluminescentie via de tijdgecorreleerde single-photon counting-techniek (TCSPC). De fotoluminescentie-relaxatiedynamiek wordt sneller bij hogere temperaturen (figuur 2a), wat kan worden toegeschreven aan de niet-stralingsvervalprocessen. De meting van de fluorescentiespectra van de CD-film als functie van de temperatuur van cryogeen tot kamertemperatuur werd uitgevoerd (figuur 2b). Bij een stijging van de temperatuur vertoont de intensiteit van de fluorescentie een herhaalde afname.
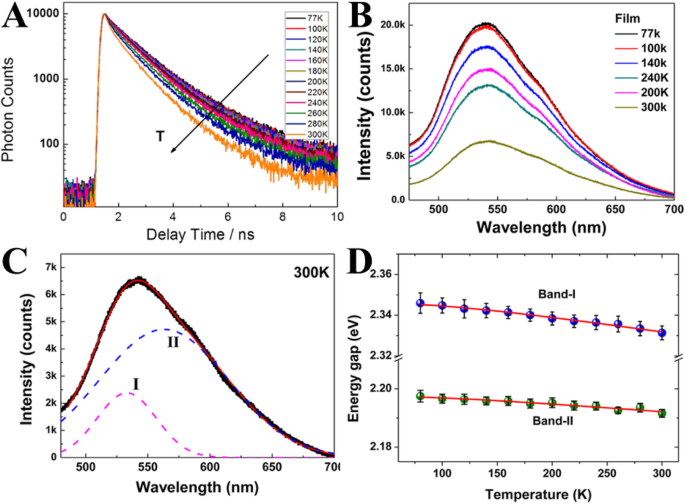
een Tijdsopgeloste fotoluminescentiemetingen als functie van de temperatuur. b Fluorescentiespectra van CD-film als functie van temperatuur. c Fluorescentiespectra bij 300 K aangepast door een twee-Gauss-functie. d De bandbreedte van fluorescentie als functie van temperatuur. (Overgenomen met toestemming van referentie [51])
Het PL-spectrum vertoont asymmetrische pieken, daarom zouden de PL-spectra bij elke temperatuur goed kunnen worden aangepast door een twee-Gauss-functie getoond in figuur 2c. De hoge energieband, band I; de lage energieband, band II (Fig. 2d).
Verder werd de energiekloof (bandbreedte) van fluorescentie geïnspecteerd als een functie van temperatuur (figuur 2d). De totale bandbreedte wordt uitgesproken door temperatuuronafhankelijk (elektron-elektronverstrooiing) en temperatuurafhankelijk (elektron-fonon- en oppervlakte-/defectverstrooiing). De bandbreedte van band I en band II is temperatuuronafhankelijk, wat aangeeft dat elektron-elektronverstrooiing domineert in de cd's.
Daarom is het zwakke temperatuureffect in CD's in overeenstemming met het feit dat het belangrijkste interactiemechanisme elektron-elektron-interacties omvat in plaats van elektron-fonon-koppeling. Bovendien wordt een brede PL-band (> 100 nm) die gewoonlijk zelfs bij zeer lage temperatuur (77 K) wordt waargenomen, aangeduid als sterke elektron-elektroninteractie (figuur 2c). Dit resultaat is vergelijkbaar met metalen nanoclusters en verschilt van halfgeleider-QD's. Daarom speculeerden Yu en collega's dat de π-elektronen in cd's op dezelfde manier kunnen werken als vrije elektronen in de metalen nanoclusters.
Kalytsjoek et al. [89] synthetiseerde zeer lichtgevende in water oplosbare N, S-CD's door een staps hydrothermische behandeling van citroenzuur en l-cysteïne. Ze verzamelden steady-state absorptiespectra bij een breed temperatuurbereik om de temperatuurafhankelijke PL-eigenschappen van de cd's te karakteriseren. De absorptiespectra van N, S-CD's gedispergeerd in water bij temperaturen tussen 10 en 70 ° C (toenemend met 5 ° C per stap) worden getoond in Fig. 3a. Anders dan bij halfgeleider nanokristallen, veranderde de positie en de intensiteit van de absorptieband niet met de temperatuur. Vergelijkbare resultaten werden eerder gerapporteerd voor CD's die zijn gesynthetiseerd door hydrothermische behandeling van glucose in aanwezigheid van glutathion [1].
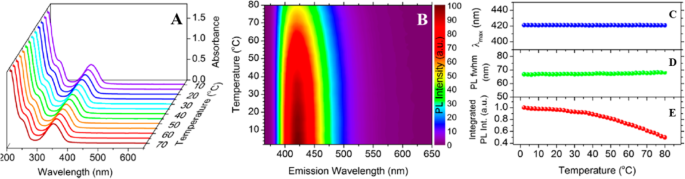
een Temperatuurafhankelijke absorptie in het temperatuurbereik van 10 tot 70 °C. b Genormaliseerde kleurengrafiek van de temperatuurafhankelijke PL-emissie bij temperaturen tussen 2 en 80 ° C met een stapgrootte van 2 ° C en excitatie bij 355 nm. Overeenkomstige temperatuurafhankelijke veranderingen in het PL-piekmaximum λmax (c ), PL fwhm (d ), en geïntegreerde PL-intensiteit (e ). (Overgenomen van referentie [89])
In hun werk toonden ze een kleurenplot van de PL-spectra van N, S-CD's verkregen bij temperaturen van 2 tot 80 ° C met een stap van 2 ° C (figuur 3b). Het verhogen van de temperatuur verminderde de PL-emissie-intensiteit met ongeveer een factor 2 zonder enige waarneembare verschuiving van de PL-emissies [89]. De positie van het PL-emissiemaximum, de PL volledige breedte op half maximum (fwhm) en de geïntegreerde PL-intensiteit werden kwantitatief bepaald bij de bestudeerde temperatuur, waarbij de resultaten in respectievelijk figuur 3c-e werden gecondenseerd.
De PL-piekpositie van N, S-CD's vertoont een zwakke temperatuurafhankelijkheid, in tegenstelling tot de meeste halfgeleider-nanokristallen, waarvan de bandafstand verandert met de temperatuur, waardoor de PL-emissieverschuiving wordt geïnduceerd. Bovendien vertoont hun PL fwhm slechts een onbeduidende verbreding (1,4 ± 1 nm) over hetzelfde temperatuurbereik (figuur 3d), wat aangeeft dat de PL-piek van de N, S-CD's verwaarloosbare thermische verbreding vertoont. Om de niet-stralingsrelaxatieprocessen die optreden in de CD's te karakteriseren, analyseerden ze de uitdoving van de geïntegreerde PL-intensiteit als een functie van de temperatuur. Een grafiek van de temperatuurafhankelijke geïntegreerde PL-intensiteit voor N, S-CD's wordt getoond in Fig. 3e, met de waarden genormaliseerd naar de intensiteit bij 2 ° C, waaruit blijkt dat de intensiteit monotoon afneemt over het bestudeerde temperatuurbereik, met die bij 80 °C is ongeveer de helft van die bij 2 °C. Op basis van deze resultaten werd de activeringsenergie van thermische quenching voor de CD's bij temperaturen tussen 2 en 80 °C geschat op 17,0 ± 0,7 meV met behulp van de Arrhenius-formule voor N, S-CD's, wat dicht bij de waarde ligt die is gerapporteerd door Yu et al. [51].
Bovendien, Kalytchuk et al. [89] onderzocht de emissiedynamiek van CD bij verschillende temperaturen. Figuur 4a-c toont de sterke temperatuurafhankelijkheid van hun in de tijd opgeloste PL-emissie, met tijdopgeloste emissiespectroscopiegegevens voor drie verschillende temperaturen. Transiënte PL-emissiekaarten van cd's werden verkregen in het spectrale gebied tussen 375 en 650 nm bij 2, 50 en 80 ° C, zoals weergegeven in Fig. 4a-c. Er is een duidelijke afname in PL-verval naarmate de temperatuur stijgt, wat suggereert dat de CD's bevredigende eigenschappen hebben voor PL-levensduurgebaseerde temperatuurwaarneming. Het is belangrijk op te merken dat spectraal uniform single-exponentieel verval werd waargenomen over de emissieprofielen van de stippen bij alle bestudeerde temperaturen, wat aangeeft dat recombinatie plaatsvindt via zeer vergelijkbare en zeer emissieve kanalen over het hele cd-ensemble. De PL-dynamiek van de cd's is misschien wel hun meest veelbelovende kwaliteit met betrekking tot toepassingen voor temperatuurdetectie. Met name de temperatuurgevoeligheid van cd's maakt ze tot PL-levensduur nanothermometers.
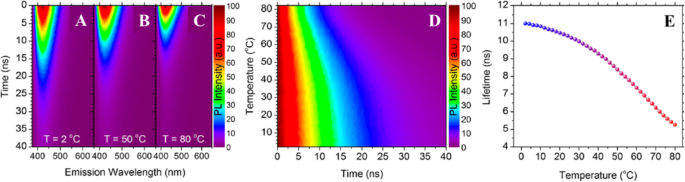
Tijdsopgeloste temperatuurafhankelijke PL-emissie van cd's. Genormaliseerde kleurengrafieken met in de tijd opgeloste PL-emissiekaarten voor cd's op a 2 °C, b 50 °C en c 80 °C. d De genormaliseerde kleurengrafiek van in de tijd opgeloste PL-intensiteit bij het PL-emissiemaximum (λem =421 nm) bij temperaturen tussen 2 en 80 ° C. e Geëxtraheerde PL-levensduren uitgezet als functie van de temperatuur in het bereik van 2-80 ° C. (Overgenomen van referentie [89])
De variatie van de PL-levensduur van de CD's bij temperaturen tussen 2 en 80 °C is grondig onderzocht; Fig. 4d, e toont in de tijd opgeloste PL-gegevens verzameld bij het PL-emissiemaximum van de stippen als een functie van de temperatuur. Een kleurengrafiek van voorbijgaande PL over het bestudeerde temperatuurbereik wordt weergegeven in Fig. 4d, waaruit blijkt dat het verhogen van de temperatuur leidt tot een monotone verkorting van het schijnbare PL-verval. Alle opgenomen vervalcurven werden aangepast met behulp van een enkelvoudige exponentiële functie. Gegevens over de geëxtraheerde levensduur worden weergegeven in Fig. 4e. Naarmate de temperatuur stijgt van 2 naar 80 °C, neemt de PL-levensduur monotoon af van 11,0 naar 5,3 ns. Het temperatuurbereik waarover deze PL-levensduurgevoeligheid is aangetoond (2-80 °C) dekt zowel het fysiologisch relevante temperatuurbereik als de typische bedrijfstemperaturen van veel elektronische apparaten. De absolute pseudo-lineaire gevoeligheid van deze PL-levensduur op cd gebaseerde thermische sonde is 0,08 ns K −1 , en de maximale relatieve gevoeligheid is 1,79% K −1 bij 62 °C. De single-exponentiële pasvorm van het PL-verval van op CD gebaseerde luminescente nanosonde over het bestudeerde temperatuurbereik levert een enkele parameter op, de PL-levensduur (τ), die direct kan worden omgezet in temperatuureenheden met behulp van een kalibratiecurve. Dit is een belangrijk voordeel ten opzichte van typische halfgeleiderkwantumdots, die multi-exponentieel verval vertonen, wat hun bruikbaarheid in toepassingen met PL-levensduurmetingen beperkt.
Ze bestudeerden ook de temperatuurafhankelijkheid van de levensduur van CD's PL in fosfaatgebufferde zoutoplossing (PBS) en Dulbecco's gemodificeerde Eagle's medium (DMEM) en vertoonden vergelijkbaar gedrag.
Om de herbruikbaarheid van op CD gebaseerde luminescentiethermometers aan te tonen, werden de PL-vervalcurven van geselecteerde monsters gemeten over zeven opeenvolgende cycli van verwarming en koeling bij temperaturen tussen 15 en 45 °C (Fig. 5a).
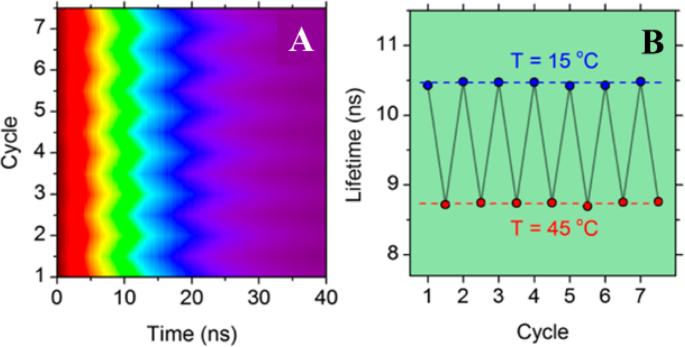
een Genormaliseerde kleurengrafiek van de omkeerbaarheid van PL-verval over zeven opeenvolgende cycli van verwarming en koeling. b Overeenkomstige thermische stabiliteit van PL-levensduur gedurende zeven cycli van verwarming en koeling tussen 15 en 40 °C. (Overgenomen van referentie [89])
In elke meetcyclus werd het PL-verval gemeten na 5 minuten thermische equilibratie. Er werd geen thermische hysterese waargenomen tijdens de verwarmings- en koelcycli, en de resulterende PL-levensduurvariatie is uitgezet als functie van de tijd in (Fig. 5b), wat aantoont dat de PL-levensduur van de cd's een uitstekende thermische stabiliteit vertoont.
Later werden verschillende CD's met temperatuurafhankelijke emissie bereid met behulp van verschillende synthesemethoden, zoals hydrothermische en solvotherme behandeling [2, 77, 92, 95, 97,98,99,100,101,102,103], warmtereflux [94, 96] en laser ablatie [14] zoals weergegeven in tabel 1. De bereide cd's vertoonden lineaire temperatuurafhankelijke fluorescentie bij de fysiologische bereiken (weergegeven in tabel 1). De fluorescentie-intensiteit van de CD's nam af met toenemende temperatuur. Bovendien zijn de omkeerbaarheid en herstelbaarheid van de fluorescentie-intensiteit in alle artikelen bestudeerd. Afbeelding 6 toont enkele algemene eigenschappen van cd's die in verschillende artikelen zijn bestudeerd.
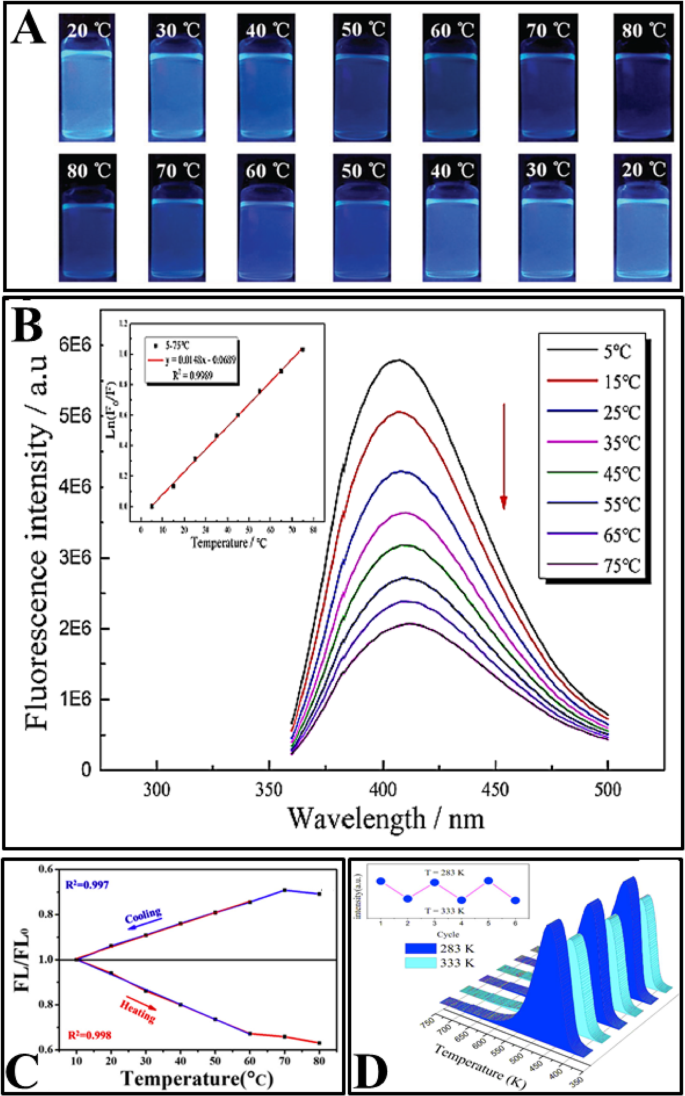
een Digitale foto's van N, S-CD's onder UV-licht (365 nm) excitatie bij verschillende temperaturen tijdens verhitting (boven) en koelprocessen (onder). (Overgenomen uit referentie [96]). b Fluorescentie-emissiespectra van N, S co-gedoteerde CD's gemeten in het bereik van 5-75 °C (van boven naar beneden) wanneer geëxciteerd bij 340 nm, inzet:de overeenkomstige lineaire regressie van de temperatuur versus Ln (F /F 0 ). (Overgenomen uit referentie [97]). c FL/FL0 -temperatuurgrafieken van MnOx-CD's tijdens koel- en verwarmingsprocessen. (Overgenomen uit referentie [103]). d Omkeerbare temperatuurafhankelijkheid van de PL van de cd-oplossing. (Overgenomen van referentie [102])
Nguyen et al. [14] gesynthetiseerde koolstofpunten (CD's) met behulp van femtoseconde laserablatie van grafietpoeder in ethyleendiamine. Overvloedige functionele groepen werden gevormd op het oppervlak die meerdere oppervlaktetoestanden op het oppervlak vormen en resulteren in de multi-emissie van CD's. Ze onderzochten de fluorescentie-afhankelijke temperatuurgevoeligheid van cd's met behulp van steady-state fluorescentiespectra. De temperatuurafhankelijke emissiespectra van de CD's bij 320 nm-excitatie worden getoond in Fig. 7a. Beide fluorescentie-intensiteiten van 400- en 465 nm-pieken nemen geleidelijk af met de temperatuurstijging als gevolg van de thermische activering van niet-stralingsvervalroutes. De piekintensiteiten veranderen lineair met een temperatuur variërend van 5 tot 85 ° C (figuur 7b). Temperatuurgevoelige cd's vertoonden een verandering in fluorescentie-intensiteit van 3,3 en 2,1% per °C voor respectievelijk 400 en 465 nm pieken. Dit geeft aan dat de cd's kunnen worden gebruikt als een conventionele, op intensiteit gebaseerde temperatuursensor met een hoge gevoeligheid.
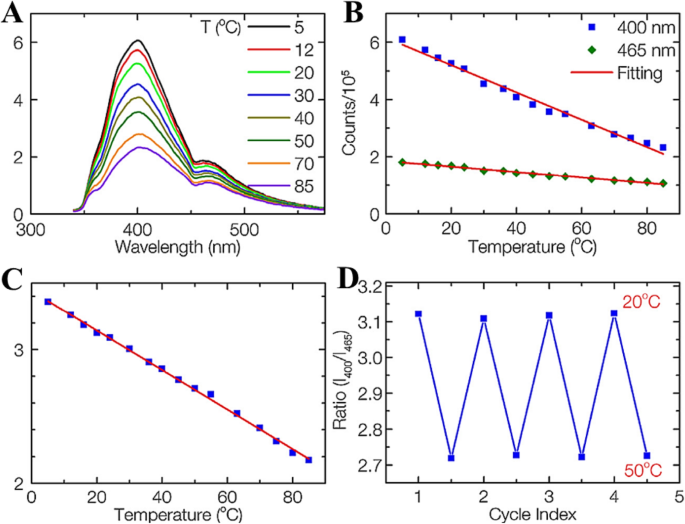
een Emissiespectra van cd's opgenomen van 5 tot 85 °C, geëxciteerd bij 320 nm. b Fluorescentie-intensiteiten van de 400 en 465 nm pieken versus temperatuur. c De verhouding van de 400 nm over de 465 nm pieken als functie van de temperatuur. d Onderzoek naar temperatuuromkeerbaarheid van cd's tussen 20 en 50 °C. (Overgenomen van referentie [14])
Opmerkelijk is dat de unieke multi-emissie-eigenschap de cd's veelbelovende fluoroforen maakt voor ratiometrische fluorescerende temperatuursensoren. De verhouding van de twee fluorescentie-intensiteiten bij 400 en 465 nm (320 nm excitatie) versus temperatuur wordt getoond in Fig. 5c. There is a very good linear relationship between the intensity ratio and temperature in a wide temperature range from 5 to 85 °C (R 2 =0.998). Thermal linearity is advantageous since it makes the correlation between the peak-intensity ratio and temperature straightforward and meanwhile provides a constant thermal sensitivity along with the entire dynamic range. The temperature-sensitivity of CDs is determined to be 1.48% °C −1 , which is comparable with that of other materials. It should be noted that the temperature response range of CDs is much wider than those of other reports on dual-emission temperature sensors and covers both the physiological temperature for biology studies and the working temperature for many electronic devices. Besides 320 nm excitation, the CDs also work at other excitation wavelengths, such as 340 and 365 nm, with the same sensitivity. Thus, the CDs can be utilized for temperature sensing in many practical applications by selected different working wavelengths.
They have shown that the ratiometric temperature sensor was reversible between 20 and 50 °C, four cycles and photostable (when the intensity of the power source changed) as shown in Fig. 7d. This result suggests that the CDs sensing system is stable and robust with any changes in sample concentration, excitation, or detection efficiency.
Increasing temperature is not always accompanied by PL quenching; however, it could show enhancement of the PL as well. Macairan et al. [29] showed the PL enhancement of dual-fluorescent carbon dots with increasing temperature. They prepared biocompatible dual-fluorescing carbon dots CDs in a one-step microwave assisted-reaction using formamide and glutathione. They found that following excitation at 640 nm, the fluorescence intensity and PL integrated area increase over the range of 5–60 C by a factor of 3.5 observed over the entire analysis range and the temperature (Fig. 8a). As shown in Fig. 8b, a linear response (R 2 =0.999) is observed over the entire analysis range and the temperature sensitivity was determined to be as high as 3.71% C −1 .
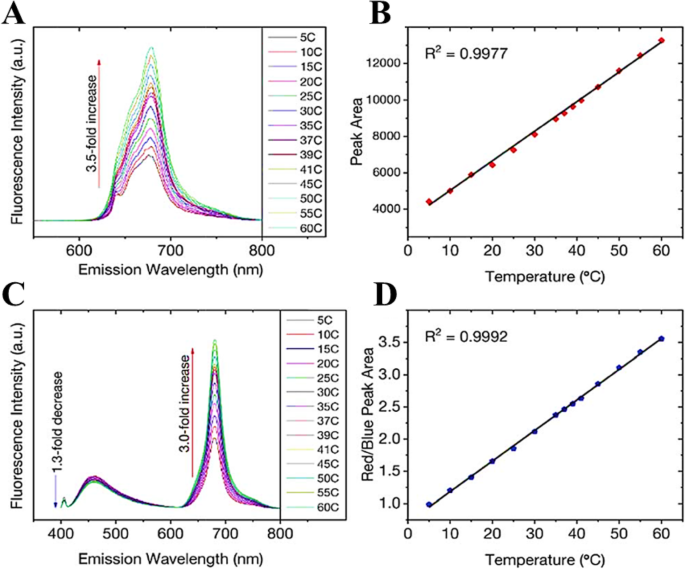
een Excitation at 640 nm yields a 3.5-fold increase in fluorescence intensity and the corresponding integrated area is plotted in b showing a linear response over the range of 5–60 °C. c Changes in the fluorescence spectra of the CDs (λex =405 nm) as a function of temperature over the entire range. A 1.3-fold decrease is noted for the blue fluorescence in contrast to the 3-fold increase for the red counterpart. d The ratio of the integrated areas of the red and blue fluorescence components are plotted as a function of temperature showing a linear increase over the entire temperature range. (Reproduced from reference [29])
The temperature-dependent fluorescence was also studied following excitation at 405 nm. Interestingly, the blue and red fluorescence bands are not equally sensitive to the change in temperature. With increasing temperature, the fluorescence intensity (and the corresponding integrated area under the curve) of the blue component shows a very slight decrease in contrast to the red component, which significantly increases (Fig. 8c). These observations are noted over the range of 5–60 °C where the blue emission decreases by a factor of 1.3 in contrast to the red emission, which increases by a factor of 3.0.
As shown in Fig. 8d, the ratio of red to blue fluorescence increases with temperature, and a highly linear response is triplicate on 3 unique samples and the linear plot reflects the observed with an R 2 =0.998. These analyses were repeated in an average of these measurements, which have small deviations at each temperature. The thermal sensitivity of the CDs, over the entire temperature range, varied from 1.33 to 4.81% °C −1 , which is an improvement over previously reported carbon dot nano-thermometry systems and other dual-emitting nanomaterials such as quantum dots and metal-organic frameworks-dye composites. The thermal resolution of the CDs was calculated to be 0.048 K −1 indicating that it is indeed possible to measure small thermal changes.
Zhang et al. [98] synthesized CDs that have temperature-responsive characteristics in the range of 25–95 °C, and they have excellent sensitivity and remarkable reversibility/recoverability (Fig. 9a). CD/epoxy composites were further prepared by uniformly doping CDs into an epoxy resin. First, 5 μg of the CDs were dissolved in 50 μL of triethylenetetramine (TETA). Then, 350 μL epoxy resin was added to the mixed solution and fully mixed by high-speed stirring. The resulting composite showed significantly enhanced temperature response.
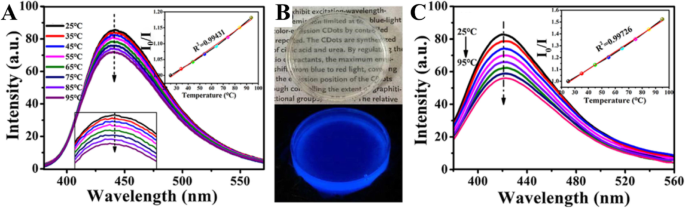
een Temperature dependence of the CD emission. b CD/epoxy composites. c Temperature dependence of the emission of the CD/epoxy composites. (Reproduced from reference [98])
Epoxy resin is a common thermosetting resin and is widely used to package LED chips. Figure 9b shows optical micrographs of CD/epoxy composite discs of approximately 2 cm in diameter and 8–10 mm in thickness. The cured CD/epoxy composites are transparent, and their fluorescence emission spectra are shown in Fig. 9c. The emission peak of the CD/epoxy composite is blue-shifted by approximately 10 nm compared to that of the CDs’ solution. Notably, the temperature response of the composite is significantly improved. In the temperature range of 25–95 °C, the fluorescence intensity decreases by 35% with increasing temperature, which is more than twice that of the solution state, and the linear results are more stable. The linear equation satisfies I 0 /Ik =0.0074 [°C] + 0.80454 (R 2 =0.99724), where I 0 en ik are the fluorescence intensity of the CD/epoxy composite before and after the temperature rise, and the excitation wavelength is 360 nm. The blue shift of the emission peak and the enhancement of the temperature response characteristics may be due to changes in the dielectric constant of the environment in which the CDs are located. The composite has a wide temperature detection range, and its excellent sensitivity and stability make it suitable for use as a temperature sensor based on a fluorescent nanomaterial in a variety of environments.
Mechanism of Thermo-sensing
Up to now, there is no well-established mechanism for explaining the thermo-sensing behavior of carbon dots. Some reports attribute the mechanism to the thermal activation of non-radiative channels of surface (trap/defect) states. The general picture is that the non-radiative channels were not activated at low temperatures, so the excited electrons could emit photons radiatively. On the contrary, as the temperature increases, more non-radiative channels became activated, and excited electrons got back to the ground state by non-radiative processes, leading to the decreasing fluorescence intensity [2, 95, 99, 100, 103]. The mechanism of CDs emissions with heating/cooling is shown in Fig. 10.
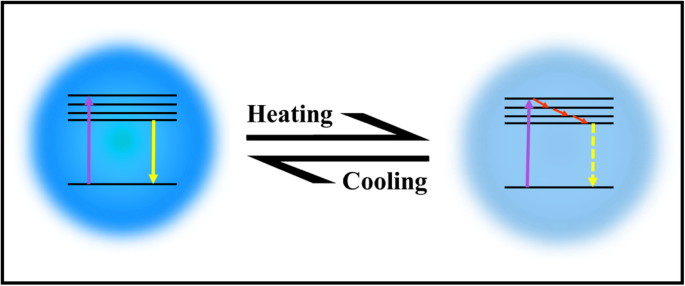
Schematic illustration of CDs responding to temperature changes
To better understand the thermodynamics of the CD emission processes, Kalytchuk et al. [89] correlated the radiative (\( {\tau}_r^{-1} \)) and nonradiative ( \( {\tau}_{nr}^{-1} \)) recombination rates of a CD sample with its PL quantum yield. The radiative rate is determined from the PL quantum yield (QY) and the measured recombination rate τ −1 as \( {\tau}_r^{-1} \) =QY × τ −1 . The nonradiative relaxation rate \( {\tau}_{nr}^{-1} \) is expressed as \( {\tau}_{nr}^{-1} \) =τ −1 - \( {\tau}_r^{-1} \). The PL QY of CDs at various temperatures was calculated from their temperature-dependent absorption and integrated PL intensity together with the PL QY determined at room temperature. Both radiative and nonradiative recombination rates derived from time-resolved PL measurement data are plotted as functions of temperature in Fig. 11. The radiative recombination rate is greater than the correspondent nonradiative rate up to 70 °C and does not vary appreciably at temperatures between 2 and 80 °C, remaining in the range of (0.74–0.82) × 10 6 s −1 . In contrast, there is a pronounced (almost 7-fold, from 0.16 × 10 6 to 1.12 × 10 6 s −1 ) monotonic increase in the rate of nonradiative recombination by increasing the temperature from 2 to 80 °C. Temperature-dependent crossover of the radiative and nonradiative rates occurs at 70 °C, at which temperature the PL QY is 50%. These results suggest that the temperature activation of PL quenching in their CDs is primarily caused by the activation of nonradiative relaxation channels [89].
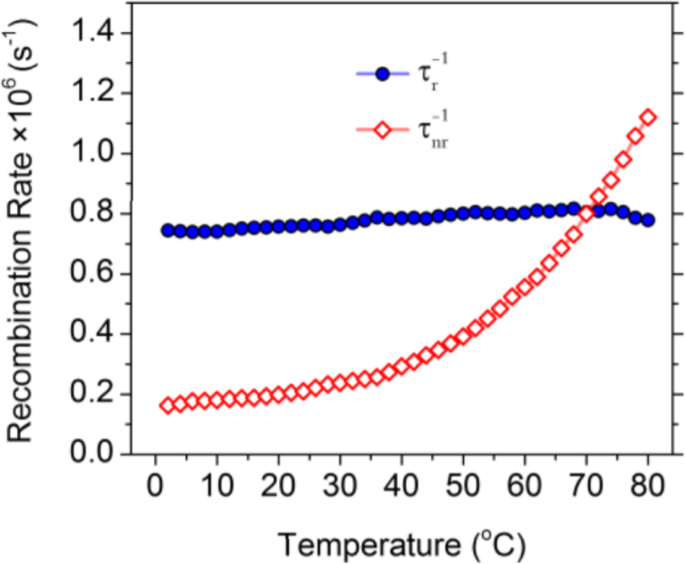
Radiative (solid symbols, blue color) and nonradiative (hollow symbols, red color) recombination rates for CDs plotted against the temperature for temperatures of 2–80 °C. (Reproduced from supporting information of reference [89])
Guo et al. [102] ascribed the thermal-quenching of their prepared carbon dots not just to activation of the nonradiative decay process, but also to the occurrence of nonradiative trapping with increasing temperature. They measured the temperature-dependent decay lifetimes of the CDs and shown in Fig. 12a. The data were collected by monitoring emission maximum as a function of the temperature under the 320 nm laser excitation. The result shows that the PL lifetime drops from 15.03 to 11.70 ns with the temperature increasing from 283 to 343 K, which could be ascribed to the occurrence of nonradiative decay processes. Besides, the PL relaxation dynamics of the CDs reveal multi-exponential decay with temperature increasing, which suggests the photoexcited carriers following the complicated relaxation processes. The occurrence of non-radiative trapping will be increased with rising temperature, and this could be quantitatively analyzed by the Arrhenius plot of the integrated PL intensities as:
$$ I={I}_0/\left[1+\mathrm{a}\ \exp\ \left(\hbox{-} {\mathrm{E}}_a/\mathrm{kT}\right)\right] $$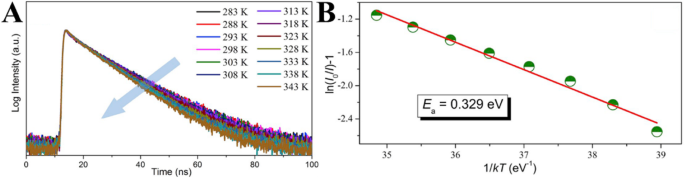
Temperature-dependent decay curves of CDs solution (a , λex =350 nm, λem =450 nm); the dependence of ln[(I 0 /Ik T )-1] on 1/kT CDs solution (b ). (Reproduced from reference [102])
waar E een is the activation energy, k is the Boltzmann constant, and a is een constante. Figure 12a displays the plotting of the emission intensity with respect to 1/T , where the value of activation energy (E een ) is calculated to be 0.329 ± 0.02 eV. In order to probe the reason for thermal quenching of CDs emission process, the radiative (V r ) and nonradiative (V nr ) recombination rates of CDs were determined from the lifetime (τ*) and quantum yield (QY) as:
$$ {\tau}^{\ast }=\frac{1}{V\mathrm{r}+V\mathrm{nr}};\mathrm{QY}=\frac{V\mathrm{r}}{V\mathrm{r}+V\mathrm{nr}} $$The QY of CDs at various temperatures was calculated from their temperature-dependent absorption and integrated PL intensity with the QY determined at room temperature. They have noticed that the radiative rates have a slight decline when the temperature rises from 283 to 343 K; at the same time, the nonradiative recombination rates have gradually increased by about 2-fold. These results further indicate that the temperature-activated PL quenching in CDs is mainly due to the activation of nonradiative relaxation channels [102].
Other groups used microscopic and spectroscopic techniques to understand the mechanism of thermos-sensing of carbon dots.
Wang at el. used TEM and UV–Vis spectra to study the temperature-responsive PL behavior of prepared CDs. As shown in Fig. 13a, the CDs display no change in the UV–Vis spectra upon increasing the temperature from 20 to 80 °C. However, it was found that the average diameter of CDs increased from 2.6 ± 0.2 nm at room temperature to 4.4 ± 0.2 nm at 80 °C (Fig. 13b). Thus, increasing the temperature, the aggregation of as-prepared CDs occurred which caused the obvious fluorescence quenching [1].
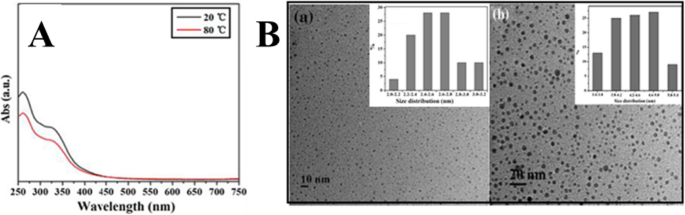
een UV–Vis absorption spectra of CDs in aqueous solution under 20 and 80 °C. b the TEM image of CDs in aqueous solution (a ) at room temperature, the average size was 2.6 ± 0.2 nm (b ) at 80 °C, and the size increased up to 4.4 ± 0.2 nm. (Reproduced from reference [1])
Hij et al. [101] reported that the hydration particle size of their CDs emerges as larger with the increase in the temperature (Fig. 14a), which indicates that the temperature rise gives rise to the aggregation of the CDs, eventually results in the fluorescence quenching. Nonetheless, with the decline in the temperature, the hydration particle size of CDs starts declining (Fig. 14b), which indicates that the cooling has the potential of causing CDs to depolymerize [101].
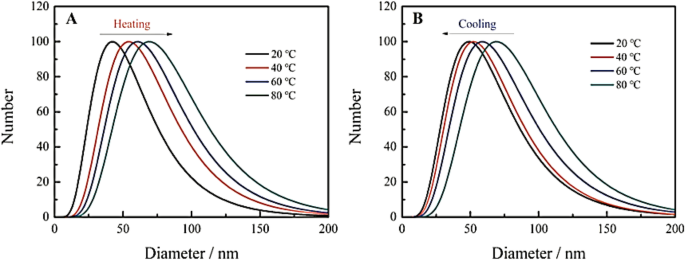
Change of hydrated particle size of carbon dots during heating (a ) and cooling (b ). (Reproduced from reference [101])
Another group such as Cui et al. also attributed the fluorescence quenching to the aggregation of CDs. They also tried to apply the undoped CDs synthesized using only acrylic acid as a precursor in temperature sensors. Unfortunately, undoped CDs possessed weaker quenching effects under the same temperature elevation than doped CDs [92].
Yang et al. [94] in their work proposed two key factors concerning the temperature-dependent PL property of the N-CDs, including (i) surface functional groups and (ii) hydrogen-bonding interaction. To examine the effect of the first factor, the surface O-containing groups, another control experiment was conducted by treating N-CDs (4.0 mL) with a strong reducing agent NaBH4 (1.0 mL, 0.1 mol L −1 ) to remove C=O species on carbon dots surface. The obtained reduced N-CDs are denoted as r-N-CDs for brevity. Compared with N-CDs, the r-N-CDs exhibit weaker fluorescence intensity (Fig. 15a). Besides, the fluorescence intensity of r-N-CDs only decreases by 13% with temperature increasing from 20 to 80 °C (the inset in Fig. 15a) that gives a much lower temperature sensitivity, which is ascribed to the decreased O-containing groups [94].
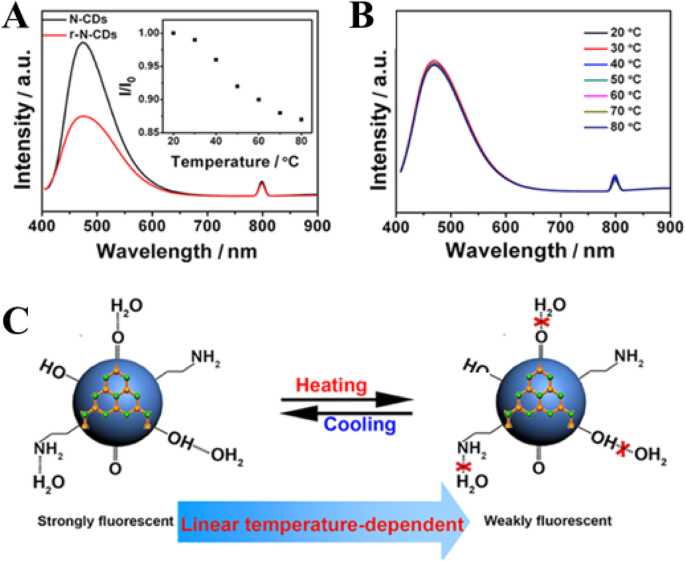
een PL spectra (excitation wavelength, 400 nm) of N-CDs (black trace) and r-N-CDs (red trace). The inset shows I /Ik 0 −T of reduced N-CDs. b PL spectra (excitation wavelength, 400 nm) of N-CDs dispersed in C2 H5 OH at various temperatures. c A schematic mechanism for the temperature-dependent fluorescence intensity of N-CDs. (Reproduced from reference [94])
The second factor, the effect of hydrogen bonding with the solvent on fluorescent behavior of N-CDs was explored. N-CDs solution (1.0 mL) was dropped on a filter paper and left to dry in the air to obtain a solid sample, which still emits bright fluorescence. However, no obvious change of fluorescence intensity of the solid N-CDs with temperature increase was observed. They also measured the fluorescence of N-CDs dispersed in ethanol. The fluorescence intensity of N-CDs in C2 H5 OH is lower than that in water and little variation of the PL intensity is observed with temperature increasing from 20 to 80 °C (Fig. 15b). Hence, the strong hydrogen bonds play a key role in the temperature-dependent PL property of the N-CDs. Figure 15c is a schematic mechanism for the temperature-dependent fluorescence intensity of N-CDs [94].
However, our group used the same experimental strategy as Yang group; in both cases, the r-CDs and e-CDs emissions were quenched linearly with increasing temperature, in the same way as the original results of their CDs (Fig. 16). Thus, our results ruled out the synergistic effects of abundant oxygen-containing functional groups and hydrogen bonds [77].
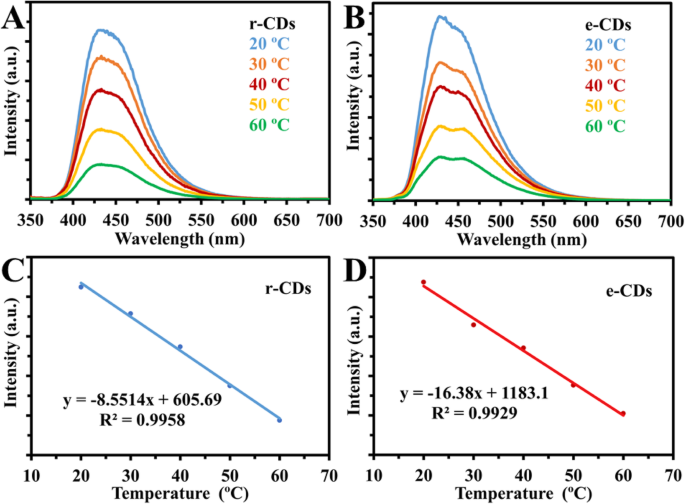
een , b Fluorescence spectra of reduced CDs (r-CDs) and CDs in ethanol (e-CDs) at temperatures (20 to 60 °C). c , d Linear correlation between fluorescence intensity and temperature (°C) for r-CDs and e-CDs respectively. (Reproduced from the supplementary information of reference [77])
Bioimaging in Living Cells (Thermal Imaging)
In literature, only a few articles explored the temperature-responsive fluorescent properties of CDs in biological imaging. Prior to such experimentations, in vitro cytotoxicity analysis is crucial for CDs because they make it possible to estimate the CD’s toxicity in living subjects. In vitro cytotoxicity analysis evaluates the effect or influence of the nanomaterial on cultured cells [89].
Yang et al. [94] verified that the CDs could be used as an effective thermometer in living cells; HeLa cells were washed with PBS after treatment with CDs for 6 h. Bright-blue fluorescence of the CDs in HeLa cells is observed when the temperature is 25 °C as shown in Fig. 17a. With the temperature increasing to 37 °C, the blue fluorescence becomes weaker (Fig. 17b), the fluorescence of N-CDs is recovered when the temperature was decreased to 25 °C (Fig. 17c). As a thermos-imaging in vivo, the fluorescent images of mice were collected immediately after being injected with N-CDs at different temperatures. By setting the fluorescence intensity at 28 °C as the reference (I o ), Ik /Ik o of the area where CDs were injected varies from 1.0 to 0.87 with the increase of temperature from 28 to 34 °C (Fig. 17d, e). With temperature further increasing to 43 °C, I /Ik o declines to 0.52 and the fluorescence becomes nearly undetectable (Fig. 17f). And I /Ik o can be reversibly enhanced back from 0.66 to 1.0 with the temperature decreases from 39 to 28 °C (Fig. 17g–i). All of these results indicated that N-CD could be used as an effective in vitro and in vivo nanothermometer [94].
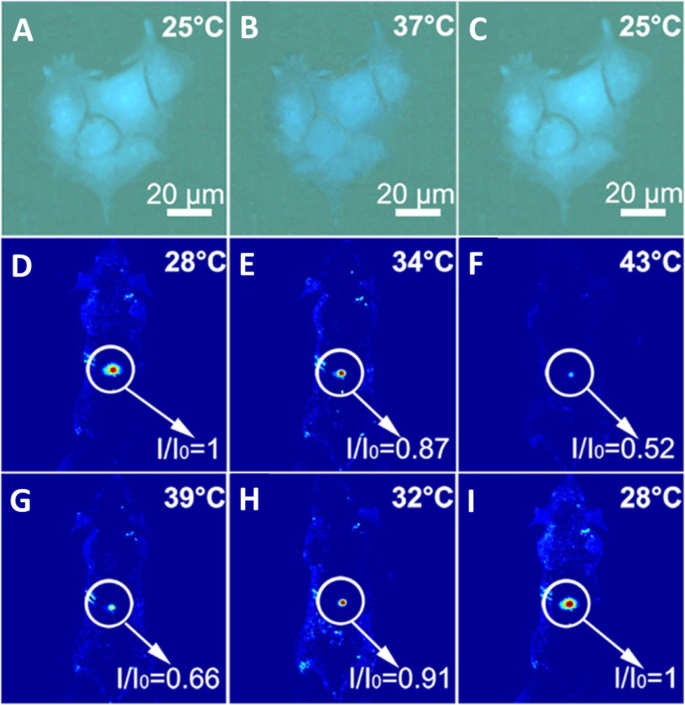
a–c Fluorescent images of a single Hela cell at 25, 37, and 25 °C after treatment with N-CDs, respectively. d –f Fluorescent photographs of a mouse given an injection of N-CDs at increasing temperatures. g –ik Fluorescent photographs of a mouse given an injection of N-CDs at decreasing temperatures. ( Reproduced from reference [94])
In an exploratory experiment, Kalytchuk et al. tested the capacity of CDs for intracellular temperature monitoring in human cervical cancer HeLa cells. Figure 18 shows the measured intracellular temperatures of HeLa cells incubated with CDs (500 μg/mL). The CDs’ PL decay curves at each temperature were highly reproducible and could be fitted with a single-exponential function at all recorded temperatures. In Fig. 18a, the recorded PL decay curves for temperatures between 25 and 50 °C (with a step size of 5 °C) are indicated by symbols, while the corresponding single-exponential fits are represented by solid lines. They were able to confirm that the PL signal in these PL lifetime measurements was derived exclusively from the CDs for the CD concentration of ≥ 100 μg/mL. The intracellular temperature in each measurement was determined from the calibration curve between PL lifetimes as the temperature increases from 2 to 80 °C. The temperatures determined in this way (T meas ) are plotted as functions of the PL lifetime in Fig. 18b. Independently, the temperature of the cell solution was determined using a calibrated reference thermometer (shown in Fig. 18b as Tset ). The temperatures reported by the luminescent CD probe and the reference detector are in good agreement. These results show that the PL lifetime of nanoprobes based on CDs can be reliably used to measure intracellular temperatures [89].
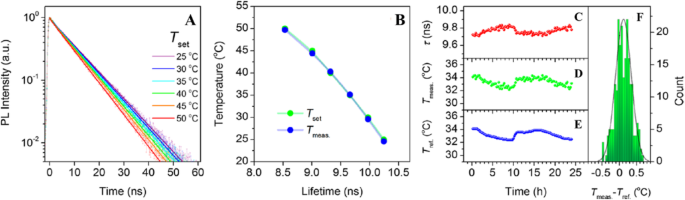
In vitro intracellular PL lifetime thermal sensing using CDs. een PL emission decays of HeLa cells incubated with CDs (500 μg/mL) at different temperatures (T instellen ). b Temperatures determined using the calibration (T meas ) and set temperatures (T instellen ) plotted against the PL lifetime. c –f Applicability of CDs for long-term remote intracellular temperature monitoring. c PL lifetimes extracted from PL transients recorded every 15 min for 24 h of HeLa cells incubated with CDs (500 μg/mL). d Temperatures determined using the calibration curve. e Temperatures measured with a reference thermometer (T ref ). v Histogram showing the distribution of temperature differences between the obtained and reference temperatures; the solid line is the distribution curve. (Reproduced from reference [89])
To further evaluate the potential of luminescent CD nanoprobes for long-term real-time temperature monitoring, PL decay profiles of HeLa cells incubated with CDs (500 μg/mL) every 15 min for 24 h were recorded. The PL lifetimes extracted from the measured PL decay values during this period were then plotted as functions of time, as shown in Fig. 18c. Using these results, the temperature variation over time was calculated using the calibration curve, as shown in Fig. 18d. In addition, the sample’s temperature at each measurement point was determined using a reference thermometer with a temperature reproducibility of 60 mK. The temperature determined with the reference thermometer is plotted in Fig. 18e, which shows that there was excellent agreement between the measured and reference temperatures. The high accuracy of the PL-based temperature measurements is further demonstrated by statistical analysis of the differences between the measured and real (reference) temperatures (Fig. 18f). Using these data, the absolute average accuracy of temperature detection by the presented method was calculated to be 0.27 °C. This experiment confirms the potential of CD-based thermal probes in biological systems [89].
Macairan et al. [29] displayed that the prepared CDs can be used for thermal sensing inside cells using intensity and ratiometric approaches. HeLa cells treated with CDs were allowed to equilibrate at 32, 37, and 42 °C (Fig. 19). Excitation at 640 nm was used to selectively monitor the red fluorescence of the CDs in the cells. The thermal changes could be due to changes in intracellular concentration or not correlate with a change in intensity (λex + 640 nm). This could be due to changes in intracellular concentration or localization of the CDs at higher temperatures. Thus, simply relying on changes in fluorescence intensity leads to accurate intracellular thermal sensing.
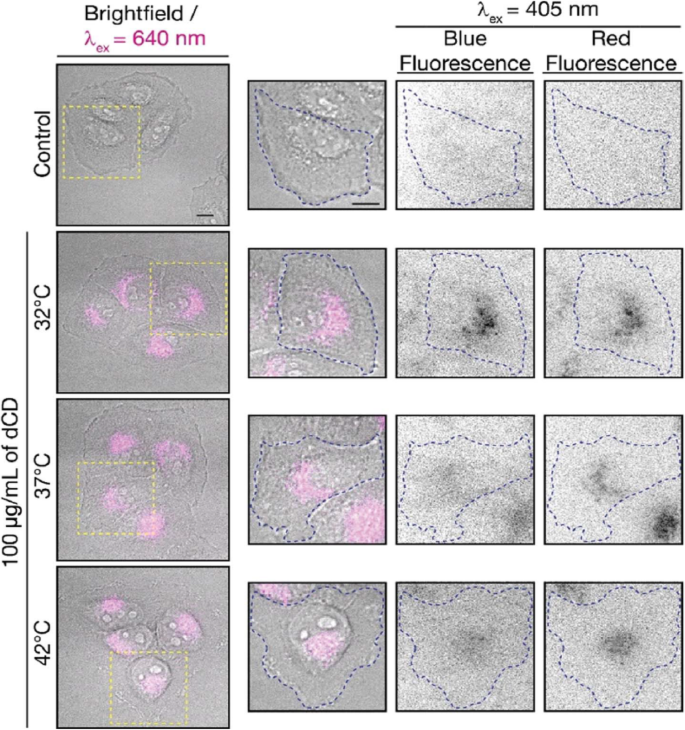
Fluorescence microscopy images of CD-treated HeLa cells. Fluorescence signals from the CDs (λex =640 nm; left and 405 nm; right) fluorescence ratios are 1.8 at 32 °C, 2.0 at 37 °C, and 2.3 at 42 °C. The control shows untreated HeLa cells at 42 °C with no fluorescence signal as expected. (Reproduced from reference [29])
In contrast, these limitations are excluded using the ratiometric approach. The CDs maintain dual blue and red fluorescence in cells following excitation at 405 nm, as previously observed for the colloidal dispersions (Fig. 19). The red-to-blue ratio increases with increasing temperature, with values of 1.8 at 32 °C, 2.0 at 37 °C, and 2.3 at 42 °C. The ratiometric relationship of the red-to-blue fluorescence of the CDs highlights the advantage of ratiometric temperature sensing in the development of fluorescent nano-thermometry probes. The relative red-to-blue emission ratio remains unaffected, regardless of the amount of CDs taken up by the cells, which can be affected by various factors such as confluency and is not concentration-dependent. Lastly, the CDs have shown fluorescence reversibility with respect to changes in intracellular temperature. Following incubation in HeLa cells, they were subjected to a heating/cooling cycle from 32/42/32 °C. This emphasizes the robustness of the proposed CD-nanothermometer and these findings further demonstrate the fluorescence reversibility [29].
Confocal laser scanning microscopy was used to thermal image colon cancer cell HT-29 using N, S, and I-doped CDs, as shown in Fig. 20 [100]. The fluorescent spots were temperature-dependent as shown in Fig. 20g–i, as the most intense is at 15 °C, while the weakest point was at 35 °C. Interestingly, the fluorescence spots were reversible, and the spots were very photostable after 20 min of continuous excitation [100]. Shin et al. [96] also used confocal laser scanning microscopy for Hela cells, shown in Fig. 20e–g.
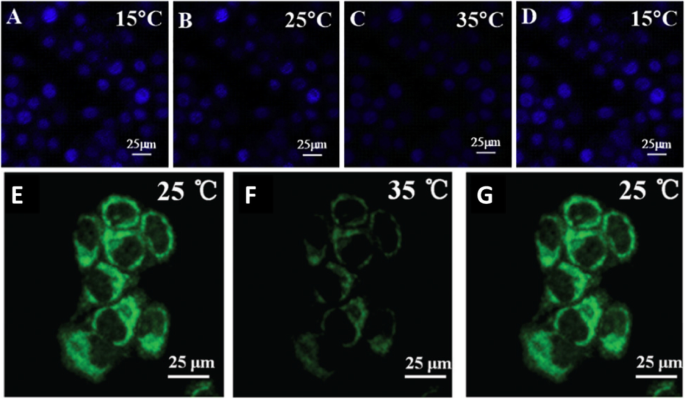
a–d Are confocal microscopy images of N, S, I-CDs-colon cancer cell HT-29 with corresponding fluorescence field at 15, 25, 35, and 15 °C, respectively. (Reproduced from reference [100]). e –g Confocal microscopy images of N, S-CDs-stained cells with corresponding fluorescence field at 25, 35, and 25 1C, respectively. (Reproduced from reference [96])
Li et al. 103 prepared a nanocomposite composed of MnOx-CDs to be used as a nano thermos responsive fluorophore for biological environments. HepG2 cells were incubated with MnOx-CDs at different culture temperatures. As illustrated by confocal laser scanning microscopy (excited at 405 nm), the blue luminescence of the MnOx-CDs in the HepG2 cells is weak when the environmental temperature was 40 °C (Fig. 21a), and the blue luminescence of the MnOx-CDs in HepG2 cells enhances as the temperature decreased to 30 °C (Fig. 21b) even to 20 °C (Fig. 21c). Due to the temperature-responsive properties, the as-synthesized MnOx-CDs can be readily applied in the biomedical fields like bioimaging and photothermal therapy in cancer treatment [103].
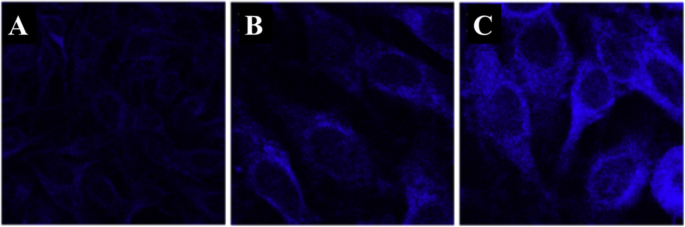
Confocal laser scanning microscopy images (excited at 405 nm) of MnOx-CDs in HepG2 cells at 40 °C (a ), at 30 °C (b ), and 20 °C (c ). (Reproduced from reference [103])
Conclusions and Future Perspective
Carbon nanodots exhibit unique properties to be exploited for nanothermometry, such as thermal-sensitivity, low-cost, and photostability. Flexible surface modification and facile preparation will pave the way to establish an enormous number of thermal sensitive nanomaterials for a variety of applications. The overall trends in thermo-sensing nanomaterials are aimed at enhancing photostability and thermal-resolution with using low-cost and safe materials. CDs can be classified as a new generation of thermometer that can fulfill these requirements and can be used for biomedical thermometry applications, such as temperature monitoring during hyperthermia treatment. Facile-preparation protocols, biocompatibility, and easy functionalization of CDs are promising criteria which make the CDs alternative next-generation nanothermometer materials. More efforts are required to promote basic research in this field. Limitations should be overcome to produce carbon dot-based nanothermometers comprising enhancing thermal sensitivity, and working in a broader range of temperature. A better understanding of the fluorescence thermal-sensing mechanism is another key issue to be able to design and manipulate the structure of CDs and enhance thermal resolution. More experiments and theoretical modeling are necessary to understand the correlation between the methods of fabrication of CDs with their thermal behavior.
Nanomaterialen
- Wat is er nieuw in materialen voor 3D-printen:recente voortgang bijhouden
- MIT-onderzoek om een nieuwe standaard te zetten voor levenscyclusanalyse
- Nieuwe reeks antivirale technologieën voor een verscheidenheid aan materialen
- Materialen:nieuwe zelfbindende LSR voor overmolding op pc, PBT
- Materialen:gloeitips voor amorfe polymeren, deel 2
- Deel 3 Materialen:gloeitips voor semikristallijne polymeren
- Materialen:met glas en koolstofvezel versterkte PP voor auto's
- DARPA werkt aan nieuwe generatie materialen voor hypersonische voertuigen
- Nieuwe kristallen voor de volgende generatie displaytechnologieën
- Trelleborg kondigt nieuwe distributeur voor gereedschapsmaterialen aan
- Nieuwe Solvay-materialen, processen ontworpen voor industrialisatie