Grafeenfamiliemateriaal bij botweefselregeneratie:perspectieven en uitdagingen
Abstract
We zijn getuige geweest van overvloedige doorbraken in onderzoek naar de bio-toepassingen van grafeenfamiliematerialen in de huidige jaren. Vanwege hun grootte op nanoschaal, groot specifiek oppervlak, fotoluminescentie-eigenschappen en antibacteriële activiteit, hebben grafeenfamiliematerialen een enorm potentieel voor botweefselengineering, medicijn- / genafgifte en biologische detectie / beeldvorming. In deze review kijken we terug op recente vooruitgang en prestaties in grafeenonderzoek, en analyseren en bespreken we kritisch de bioveiligheid en haalbaarheid van verschillende biomedische toepassingen van grafeenfamiliematerialen voor botweefselregeneratie.
Inleiding
Slachtoffers van ernstige maxillofaciale infectie, trauma, tumor en aangeboren misvorming die lijden aan kaakbotdefecten, vereisen gewoonlijk langdurig herstel. In tegenstelling tot veel andere weefsels heeft het bot een uitstekend vermogen om te regenereren wanneer het beschadigd is [1, 2]. Het beperkte zelfherstellende vermogen van het menselijk skelet maakt de reconstructie van botdefecten die groot genoeg of kritiek groot zijn echter een belangrijke uitdaging voor klinische therapie [3]. In sommige gevallen hebben de ernstige patiënten zelfs uitgebreide botvergrotingsoperaties nodig. De huidige therapieën voor botregeneratie bestaan uit autograft, allograft en xenograft [4]. Autoloog bot wordt beschouwd als het "gouden standaard" bottransplantaatmateriaal, met de mogelijkheden van osteoconductie, osteo-inductie en osteogenese, ook zonder immunogeniciteit. Maar de redenen waarom autograft nog steeds beperkt is voor gebruik in de kliniek, zijn het risico op donorinfectie en de lange hersteltijd [5]. Allograft, verkregen van een ander individu, wordt vaak beschouwd als de op één na beste optie. Maar het gebruik van allograft brengt potentiële risico's met zich mee, zoals een dramatisch verhoogd risico op infectie en immuunafstoting [4, 6]. Xenotransplantaatmaterialen, zoals met zuur verteerd gedemineraliseerd botmatrix en rundercollageen, zijn gemakkelijk te verkrijgen en te vervaardigen. Nu is xenotransplantaat een belangrijke benadering in de klinische praktijk. Maar het heeft lage osteo-inductieve capaciteiten [7]. Op dit moment zijn er in vergelijking met het bot geen heterologe of synthetische botvervangers beschikbaar die superieure of zelfs dezelfde biologische of mechanische eigenschappen hebben [5]. Hoewel deze therapieën nuttig zijn gebleken, lijden ze aan inherente uitdagingen. Daarom moet een adequate botregeneratietherapie nog worden onderzocht en ontwikkeld. Toegegeven, onderzoek naar botweefselmanipulatie en regeneratieve geneeskunde openen wegen voor het verbeteren van de resultaten en het versnellen van het herstel van patiënten met botdefecten [8]. Weefsel-engineered botconstructies hebben het potentieel om de vraag te verlichten die voortvloeit uit het tekort aan geschikte autograft- en allograftmaterialen voor het verbeteren van botgenezing [9]. Om het botvolume in gebieden met botdefecten te vergroten, is een verscheidenheid aan methoden voor botregeneratie ontwikkeld, waaronder scaffolds [1, 6], coatings [10] en barrièremembranen voor geleide botregeneratie (GBR) [11, 12].
Momenteel heeft het potentieel van grafeenfamiliematerialen enorme aandacht getrokken als 2D-vlakke coating of 3D-poreuze steigers voor de differentiatie van verschillende soorten stamcellen naar neurogeen [13,14,15], chondrogeen [16, 17], myogeen [18] , adipogene [19] en osteogene lijnen [20, 21]. Materialen uit de grafeenfamilie zijn dus waarschijnlijker een kandidaat van de keuze voor de volgende materialen voor botregeneratie. Grafeen, gedefinieerd als een enkele of enkele lagen sp 2 -gehybridiseerde koolstofatomen, werd voor het eerst geïsoleerd uit grafiet door Novoselov en Geim in 2004 [22]. Met de toenemende onderzoeksinteresses worden materialen van de grafeenfamilie, waaronder grafeenoxide (GO), carboxylgrafeen (CXYG), gereduceerd grafeenoxide (rGO) en grafeenquantumdots (GQD's), uitgebreid bestudeerd. Grafeen bezit uitzonderlijk mechanische, geleidende, thermische en optische eigenschappen [23,24,25], die op grote schaal zijn toegepast in de elektronica, biotechnologie en polymeerwetenschap [26]. Erkend wordt dat geleidende materialen met veelbelovende geleidbaarheid de cellulaire activiteiten versterken en het herstel van botweefsel stimuleren [27, 28], waarbij ook goede antibacteriële activiteit wordt vertoond [29]. Grafeenoxide (GO) en carboxylgrafeen (CXYG) zijn beide derivaten van grafeen. Door de aanwezigheid van geoxygeneerde functionele groepen (epoxide-, carboxyl- en hydroxylgroepen) hebben GO en CXYG een betere dispersie in hydrofiele oplosmiddelen, wat essentieel is voor biomedische toepassingen [30, 31]. Gereduceerd grafeenoxide (rGO) kan onder bepaalde omstandigheden worden gesynthetiseerd door GO te reduceren met specifieke reductiemiddelen. Dankzij de vermindering van enkele speciale π-π chemische interacties, bezit rGO bepaalde betere fysische en chemische eigenschappen dan grafeen en GO [32, 33]. De grondstof van grafeen quantum dots (GQD's) is GO. GQD's hebben sterke kwantumbeperkingen en fotoluminescentie-eigenschappen [34]. De sterke fluorescentie van de GQD's maakt ze bruikbaar bij cellulaire beeldvorming. Vanwege de uitstekende eigenschappen van grafeenfamiliematerialen hebben ze een enorm potentieel voor medicijn- / genafgifte, biologische detectie / beeldvorming en weefselmanipulatie [35,36,37,38,39]. Er bestaan echter nog steeds uitdagingen met betrekking tot de biologische veiligheid op lange termijn en het vermogen om osteogene celdifferentiatie van grafeenfamiliematerialen te induceren. Hier bespreken we uitgebreid recente vooruitgang en prestaties op het gebied van grafeen en zijn derivaten. Tegelijkertijd analyseren we kritisch de in vitro en in vivo bioveiligheid en bespreken we de haalbaarheid van verschillende biomedische toepassingen van grafeenfamiliematerialen voor botweefselregeneratie.
Uitdagingen bij het bepalen van de bioveiligheid van grafeenfamiliematerialen
Uitdagingen bij het bepalen van de in vitro bioveiligheid
Voordat grafeenfamiliematerialen worden overwogen voor klinische proeven, moeten ze rigoureus worden beoordeeld op hun cytotoxiciteit en biocompatibiliteit [38]. "Is grafeen een biocompatibel materiaal?" Het antwoord is nog steeds controversieel. Het ruwe grafeen zonder enige functionalisering is hydrofoob en agglomereert gemakkelijk in waterige media [34, 40]. Op hydrofobe oppervlakken kan een dichte laag van niet-specifieke eiwitten water van het oppervlak verdringen en zich onmiddellijk op de materialen ophopen, wat resulteert in een immunologische herkenning van de nanodeeltjes [41]. Chemische functionalisering, inclusief oxidatie, reductie en introductie van functionele groepen, is dus een vereiste voor grafeen dat wordt gebruikt in biomedische toepassingen, die de hydrofiliciteit van grafeen verhogen. Materialen uit de grafeenfamilie met verschillende functionaliteiten, met verschillende chemische eigenschappen, oefenen verschillende toxiciteiten uit [13]. Soumen et al. ontdekte dat rGO minder toxisch was dan GO. Het was interessant om te zien dat oxidatieve stress toenam met een toenemende mate van functionele zuurstofgroepdichtheid op het rGO-oppervlak. Ze concludeerden dat de functionele groepsdichtheid op het GO-blad een van de belangrijkste factoren was bij het mediëren van cellulaire cytotoxiciteit [31]. Afgezien van oppervlaktefunctionalisering, werd de cytotoxiciteit van materialen uit de grafeenfamilie beïnvloed door tal van factoren, waaronder hun concentratie, grootte en vorm [42].
Ten eerste hebben sommige onderzoeken aangetoond dat materialen uit de grafeenfamilie dosisafhankelijke cytotoxiciteit hadden met of zonder tijdsafhankelijke cytotoxiciteit. Bijvoorbeeld Chang et al. rapporteerde dat een licht verlies van cellevensvatbaarheid werd waargenomen bij hoge concentraties GO (≥ 50 g/ml) en GO kan intracellulaire accumulatie induceren en een dosisafhankelijke oxidatieve stress veroorzaken in een epitheelcellijn van longcarcinoom (A549) [43]. Wei et al. toonde aan dat ongerepte GO de proliferatie van mesenchymale botstamcellen (BMSC's) remde bij een hoge concentratie van 10 g / ml, terwijl de proliferatie van BMSC's bij een lage concentratie van 0,1 g / ml [44]. Evenzo werd duidelijk een verminderd aantal cellen waargenomen bij 200 g/ml GO en werd een groter cytotoxiciteitseffect gemeld bij 300 μg/ml GO [45]. Wat meer is, Kim et al. vond dat de levensvatbaarheid van preosteoblasten (MC3T3-E1) licht werd beïnvloed door rGO bij concentraties <-62,5 μg/ml, maar significant (p < 0,05) nam af bij hogere concentraties (≥ 100 μg/ml) [23]. Bovendien vertoonden CXYG en GQD's beide weinig cytotoxisch potentieel bij toepassing in lage concentraties [34, 46]. Simpel gezegd, materialen uit de grafeenfamilie zijn cytocompatibel bij een lage concentratie met weinig negatieve invloed op celmorfologie, levensvatbaarheid en proliferatie, maar de concentratie is niet de enige relevante factor.
Ten tweede wordt aangegeven dat de diverse vormen, zoals lagen, nanosheets en vlokken, linten en stippen, ook bijdragen aan de complexiteit van de cytotoxiciteit van de grafeenfamilie [40]. Talukdar et al. evalueerde de cytotoxiciteit van grafeen-nano-uien (GNO's), GO-nanoribbons (GONR's) en GO-nanoplaatjes (GONP's). De CD50 waarden volgden de trend GNO's> GONR's> GONP's, wat aangeeft dat GONR's cytotoxischer waren in vergelijking met GONP's [47]. De vorm van de nanomaterialen van de grafeenfamilie is dus ook een sleutelcomponent bij het mediëren van cytotoxiciteit. Grafeen en meerwandige koolstofnanobuizen (MWNT's) hebben bijvoorbeeld verschillende vormen (platte atomaire platen voor grafeen en buisvormig voor nanobuizen), maar hun chemische samenstelling en kristallijne structuur zijn vergelijkbaar. GO vertoonde de celgroeiremmende activiteit van SK-N-SH-cellen pas bij 50 g/ml. Ter vergelijking:MWCNT's remden de proliferatie van de cel bij een lage concentratie (6,7 g/ml), wat wijst op de acute cytotoxiciteit. Voor HeLa-cellen vertoonde GO een geringe groeiremmende activiteit, zelfs bij concentraties tot 50 g / ml, terwijl MWCNT's matige cytotoxiciteit hadden op HeLa-cellen [48]. Ze waren afhankelijk van dit fenomeen op hun verschillende vorm en gevarieerde fysische/chemische manieren. Van materialen uit de grafeenfamilie werd verwacht dat ze een kleine interactie hebben met de celmembranen vanwege de platte vormen. Buisvormige vorm van MWCNT's bevorderde de penetratie van membranen, resulterend in de cytotoxiciteit [48,49,50]. Een andere belangrijke informatie is dat de cytotoxiciteit van nanogestructureerde grafeenderivaten ook afhankelijk is van het celtype, naast de afhankelijkheid van functionalisering, concentratie, grootte en vorm. Als neurale cellijn vertoonden SK-N-SH-cellen meer gevoeligheid dan HeLa-cellen voor de nadelige effecten van nanogestructureerde grafeenderivaten [48].
Ten derde speelt de grootte ook een belangrijke rol bij de bioveiligheid van materialen uit de grafeenfamilie. Yoon et al. evalueerde dat het grootteafhankelijke cytotoxische effect van grafeen-nanovlokken via een celgebaseerde elektrochemische impedantie-biosensor. Ze ontdekten dat de kleinere grafeen-nanovlokken (30,9 ± 5,4 nm) apoptose induceerden vanwege een hogere opname door cellen, terwijl de grotere grafeennanovlokken (80,9 ± 5,5 nm) die meestal op celmembranen aggregeerden, minder toxiciteit veroorzaakten [51]. Het is algemeen bekend dat cellulaire opname-eigenschappen van nanomaterialen de celproliferatie, differentiatie en uitscheiding van nanodeeltjes kunnen beïnvloeden [52]. Mu et al. werkte de waarschijnlijke grootteafhankelijke opnamemechanismen van met eiwit gecoate GO-nanosheets uit en merkte op dat grotere nanosheets (860 ± 370 nm) zich eerst op het celoppervlak hechtten, gevolgd door membraaninvaginatie, uitbreiding van pseudopodia en uiteindelijk cellen binnenkwamen, voornamelijk via fagocytose, terwijl kleinere nanosheets ( 420 ± 260 nm) kwamen de cellen voornamelijk binnen via clathrine-gemedieerde endocytose [33]. Das et al. zaai menselijke endotheelcellen van de navelstrengader (HUVEC) in 10 μg / ml GO en rGO met vellen van verschillende grootte (800 nm en 400 nm). De resultaten toonden aan dat de kleinere vellen giftiger waren dan grotere in de MTT-assay. En vervolgens werden de grotere GO en rGO (800 nm) ultrasoon behandeld om in kleinere maten (70 nm) te worden gebroken. Verhoogde cytotoxiciteit werd waargenomen na ultrasone trillingen, wat aangeeft dat de kleinere GO en rGO meer toxiciteit vertoonden [31]. Evenzo werden MCF7-cellen blootgesteld aan monsters van vier afmetingen van GO (744 ± 178 nm, 323 ± 50 nm, 201 ± -28 nm en 100 ± 10 nm). In vergelijking met de onbehandelde cellen werd in vitro geen cytotoxiciteit waargenomen, zelfs niet na 72 uur blootstelling aan de grotere GO-dispersies (744 ± 178 nm), terwijl de behandeling met 100 ± 10 nm-sized GO-dispersies resulteerde in een afname van de celproliferatie tot ongeveer 50% van de onbehandelde cellen [53]. Uit de bovenstaande resultaten is een breed scala aan maten van grafeenfamiliematerialen onderzocht, van 30 tot 860 nm. En we lijken tot de conclusie te komen dat materialen uit de grafeenfamilie van kleinere afmetingen giftiger zijn dan grotere. Maar een ander team heeft een andere standaard om de schaalgrootte van grafeen en zijn derivaten te definiëren. Dus deze conclusie is misschien discutabel. Ondertussen werd gemeld dat grafeenfamiliematerialen van nanoformaat veel veiliger waren voor biomedische toepassingen [54]. Grootte-control synthese van grafeen familie materialen moet voorzichtig worden overwogen in volgende onderzoeken.
Er wordt geconcludeerd dat de cytotoxiciteit van grafeen kritisch gerelateerd is aan de verscheidenheid aan grafeenfamilie, chemische functionalisering, concentratie, vorm en grootte. In de toekomst willen we biocompatibele apparaten maken met betere interacties met cellen, weefsels of organismen door een betere controle van concentratie en grootte, door de grafeenfamilie aan te passen met verschillende soorten functionele groepen.
Uitdagingen bij het bepalen van de bioveiligheid en biodistributie in vivo
Om verder te detecteren of grafeenfamiliematerialen biocompatibele materialen zijn en om het voorgestelde gebruik in wijdverbreide toepassingen te verbeteren, is in vivo experiment een onmisbare methode. Veel onderzoeken naar biocompatibiliteit en biodistributie van grafeenfamiliematerialen in vivo zijn bijna consistent met hun cellulaire studies. Chowdhury et al. toegepast zebravisembryo op grotere GO-dispersies en vond geen verhoogde mortaliteit van embryo's in vergelijking met de controlegroep, terwijl verminderde levensvatbaarheid van embryo's werd waargenomen in de kleinere GO-dispersies [53]. GO leidde niet tot een significante toename van apoptose in embryo, terwijl MWCNT's resulteerden in ernstige morfologische defecten in zich ontwikkelende embryo's, zelfs bij een relatief lage concentratie van 25 mg/L [48]. Deze onderzoeken gaven verder aan dat de in vivo toxiciteit sterk afhangt van de afmetingen, concentraties en vormen van grafeen en zijn derivaten. Bovendien worden grafeenfamiliematerialen meestal blootgesteld aan diermodellen via intraveneuze injectie, inhalatie of subcutane implantatie. Veranderingen in toxiciteit, algemene histologie en biodistributie variëren dus. Li et al. evalueerde de toxicologie van GO op nanoschaal bij muizen via intraveneuze injectie en ontdekte dat GO meestal werd vastgehouden in de lever, long en milt en veroorzaakte schade, chronische hepatitis en longfibrose. Een polyethyleenglycol (PEG) coating van GO (GO-PEG) zou de retentie van GO in de lever, long en milt kunnen verminderen en de acute weefselbeschadigingen kunnen verlichten [55]. Duch et al. onderzochten strategieën om het toxische effect van grafeen-nanomaterialen in de longen te verminderen, omdat ze ontdekten dat GO een hogere toxiciteit had dan geaggregeerd grafeen en Pluronic-gedispergeerd grafeen wanneer het rechtstreeks in de longen van muizen werd toegediend, wat ernstige en aanhoudende longbeschadiging veroorzaakte. De toxiciteit werd significant gematigd door de fabricage van ongerept grafeen door exfoliatie in de vloeibare fase en werd verder geminimaliseerd wanneer het werd gedispergeerd met het blokcopolymeer Pluronic [56]. Zha et al. identificeerde de korte termijn (eerste 2 weken na implantatie) en lange termijn (7 maanden) in vivo toxiciteit en prestaties van 3D grafeenschuim (GF's) of grafeenoxideschuim (GOF's) in een rattenmodel van subcutane implantatie. De bloedanalyse toonde aan dat GF's en GOF's geen merkbare hematologische, lever- of niertoxiciteit veroorzaakten na implantatie en dat er geen significante degradatie werd waargenomen na implantatie van ten minste 7 maanden. Alleen granulomen die lange tijd op de implantatieplaats bestonden, werden waargenomen. HE-gekleurde afbeeldingen vertoonden een betere in vivo biocompatibiliteit (Fig. 1) [40]. De reden waarom Zha et al. bereikten meer positieve resultaten dan andere onderzoeken, aangezien de bovengenoemde waarschijnlijk de verschillende toedieningsroutes zijn. Subcutaan experiment was de zeer directe en effectieve manier om de in vivo biocompatibiliteit van geïmplanteerde materialen [57] te beoordelen, wat een effect kan hebben op de contactpatronen, afzettingslocaties, zelfs de afbraakroutes van de grafeenfamilie nanomaterialen in vivo [58]. Het beheersen van de degradatie van composieten is van vitaal belang bij tissue engineering,
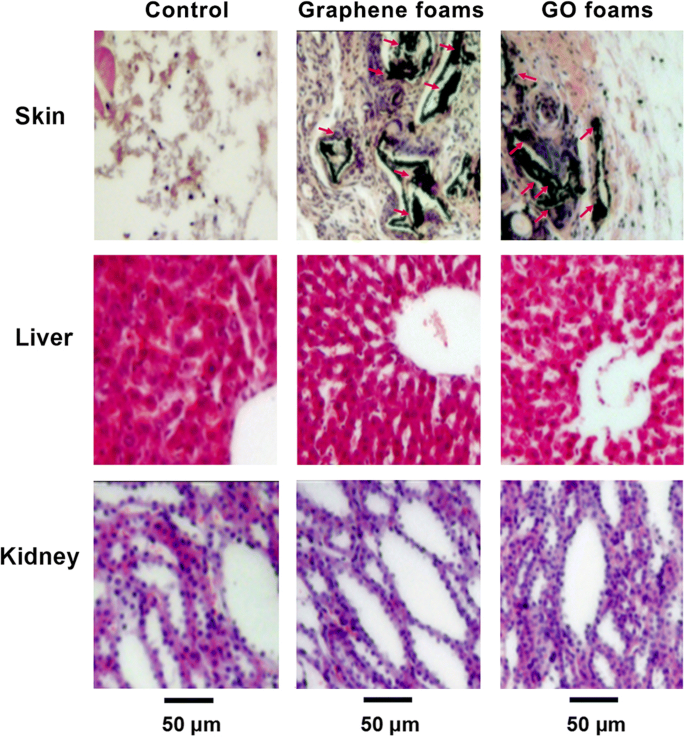
Representatieve HE-gekleurde afbeeldingen van belangrijke organen (implantatiegebied, lever en nier verzameld van de ratten) geïmplanteerd met grafeenschuim, GO-schuim of niets op dag 14 na implantatie. Er werd geen duidelijke orgaanschade of laesie waargenomen. Overgenomen van ref. [40] met toestemming van het Journal of Nanoparticle Research
Over het algemeen zijn cellulaire onderzoeken uitstekend geschikt voor een voorlopige cytotoxiciteitsanalyse, begrip van het waarschijnlijke mechanisme van interactie met cellen. Maar het is een veel gecompliceerdere micro-omgeving in vivo. Het is ook van cruciaal belang om te begrijpen hoe materialen uit de grafeenfamilie zich gedragen in vochtige corrosieve micro-omgevingen. De biocompatibiliteit van grafeenfamiliematerialen is niet-triviaal gerelateerd aan de concentratie, de variëteiten van functionele groepen, soorten grafeenfamilie, maten en vormen. Maar het mechanisme moet nog in detail en grondig worden onderzocht. De beoordeling van in vivo bioveiligheid is echter vergelijkbaar niet veel, vooral de biocompatibiliteit en biodistributie op lange termijn, waar we meer aandacht aan moeten besteden. Hoewel sommige artikelen bezorgdheid uiten over bioveiligheid, heeft de potentiële veelzijdigheid die de grafeenfamilie uniek biedt het tot een concurrerende kandidaat voor biomedische toepassingen gemaakt.
Antibacteriële activiteit van grafeenfamiliematerialen
Bothervorming en nieuwe botvorming kunnen niet volledig succesvol zijn zonder een steriele micro-omgeving van het botdefect. In feite is de behandeling van infectieus botdefect nog steeds een grote uitdaging [59]. Vanwege het grote botdefect en het infectieuze probleem is de behandeling zwaar en hebben patiënten een langdurige herstelperiode nodig. Dus het vermogen van bacterieremming van grafeenfamiliematerialen helpt veel. Er wordt aangenomen dat materialen uit de grafeenfamilie het vermogen van antibacteriën bezitten (tabel 1). Liu et al. stelde een driestaps antimicrobieel mechanisme voor, waaronder (1) initiële celafzetting op op grafeen gebaseerde materialen, (2) membraanstress veroorzaakt door direct contact met scherpe nanosheets, en (3) de daaruit voortvloeiende superoxide-aniononafhankelijke oxidatie [60]. Mangadlao et al. dacht dat het oppervlak van grafeen primair verantwoordelijk was voor antimicrobiële activiteit en niet de randen. In contact met bacteriën diende grafeen als een elektronenacceptor die het elektron wegpompte van het membraan van de bacterie, waardoor een onafhankelijke oxidatieve stress ontstond [61]. Ondertussen hebben Li et al. leverde nieuwe inzichten op voor een beter begrip van de antibacteriële werking van grafeenfilm. Ze zijn van mening dat de antibacteriële activiteit van grafeenfamiliematerialen niet voortkwam uit door reactieve zuurstofspecies (ROS) gemedieerde schade, maar via elektronenoverdrachtinteractie van microbieel membraan naar grafeen [62], terwijl Panda et al. bewees dat een synergetische invloed van niet-oxidatief elektronenoverdrachtsmechanisme en de daaruit voortvloeiende ROS-gemedieerde oxidatieve stress voor de bacteriën een verhoogde antimicrobiële activiteit van natuurlijk afgeleide GO-metaalfilms induceerde [63].
Hoewel het onzeker blijft hoe de fysisch-chemische eigenschappen van op grafeen gebaseerde platen hun antimicrobiële activiteit beïnvloeden, is het vermogen van antibacteriën van grafeenfamiliemateriaal het waard om te bestuderen en verder te profiteren van.
Grafeenfamiliematerialen bemiddelen cellen in osteogene differentiatie en bevorderen botregeneratie in vivo
Veel wetenschappers hebben erop gewezen dat grafeen niet alleen de aanhechting en proliferatie van cellen mogelijk maakt (bijv. tandpulpstamcellen [64, 65], beenmergstamcellen [8, 20, 66, 67], parodontale ligamentstamcellen [68] ], menselijke osteoblasten [69], fibroblastcellen [70], tumorcellen [43]) zonder tekenen van schijnbare cytotoxiciteit, maar kunnen ook vroege osteoblastische differentiatie van cellen induceren en een hoge mate van mineralisatie opleveren [20, 64,65,66,67, 68]. Op dit moment hebben talloze teams nauwgezet overvloedig onderzoek gedaan om nieuwe strategieën te ontwerpen voor het toepassen van grafeenfamilie-nanomaterialen als een scaffold of een additief aan de scaffold, als een coating op het substraatmateriaaloppervlak, als een geleidend botregeneratiemembraan en als een medicijnafgiftevehikel (Figuur 2). Ze probeerden grafeenfamiliematerialen te gebruiken om de bepaalde eigenschappen van het substraatmateriaal nog verder te verbeteren en een bioactief karakter te geven aan de op substraat gebaseerde composieten.
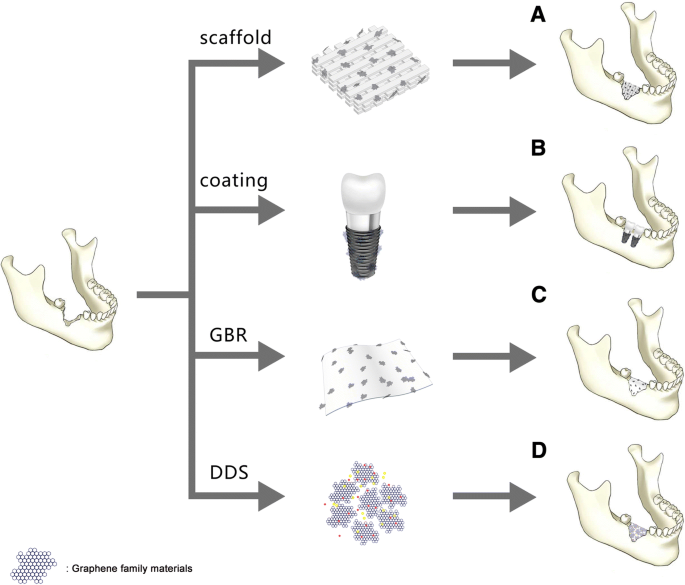
A. Grafeenfamiliematerialen als steiger of versterkingsmateriaal in steiger voor botregeneratie. B. Grafeenfamiliematerialen als coating overgebracht op het substraat voor botregeneratie. C. Grafeenfamilie als additief in geleid botmembraan. D. Materialen uit de grafeenfamilie als medicijnafgiftesysteem faciliteert botregeneratie
Grafeenfamiliemateriaal als steiger of verstevigingsmateriaal in steiger
De meest gebruikelijke strategie voor botweefselmanipulatie is het nabootsen van het natuurlijke proces van bothervorming en -regeneratie. Aan de strategie kan worden voldaan door een driedimensionale (3D) biocompatibele, biologisch afbreekbare en osteoconductieve of osteo-inductieve steiger [3]. Dit soort scaffold kan een ideale micro-omgeving bieden om de extracellulaire matrix (ECM) na te bootsen voor aanhechting, migratie, proliferatie en differentiatie van osteogene cellen, evenals voor de dragers van groeifactoren [6]. Grafeen als een veelbelovende biocompatibele steiger kan het grote oppervlak beschikbaar maken voor celverspreiding en zelfs osteogene differentiatie in het substraat [20]. 3D-grafeenschuimen die werden gebruikt als kweeksubstraten voor menselijke mesenchymale stamcellen (hMSC), leverden bijvoorbeeld bewijs dat ze in staat waren de levensvatbaarheid van de stamcellen te behouden en osteogene differentiatie te bevorderen [66]. Bovendien werd bewezen dat 3D-grafeen (3DGp) -steiger evenals 2D-grafeen (2DGp) -coating in staat zijn om de differentiatie van parodontale ligamentstamcellen (PDLSC) tot volwassen osteoblasten te induceren door de hogere niveaus van mineralisatie en opgereguleerd botgerelateerd gen en eiwitten op grafeen, met of zonder het gebruik van chemische inductoren [68].
Tegenwoordig schieten de diverse biomaterialen die als steiger dienen als paddenstoelen uit de grond. De potentieel geschikte synthetische steigers voor gebruik bij botregeneratie omvatten calciumfosfaat, zoals hydroxyapatiet (HA) [71]; β-tricalciumfosfaat (β-TCP) [72]; synthetische of biopolymeren, zoals polymelkzuur (PLA) [73], polyglycolzuur (PLGA) [74], polycaprolacton (PCL) [75], chitosan (CS) [1] en collageen [76 ]; en composieten van de bovengenoemde materialen [77, 78]. Maar nu is een van de belangrijkste zorgen de mechanische eigenschappen van steigers. Aangezien natuurlijk bot superelastische biomechanische eigenschappen vertoont met een Young's moduluswaarde in het bereik van 7-27 GPa [79], zouden de ideale scaffolds de sterkte, stijfheid en mechanisch gedrag van natuurlijk bot moeten nabootsen. Materialen uit de grafeenfamilie kunnen worden toegevoegd als versterkt materiaal in steigers met als doel de mechanische eigenschappen te versterken en de fysisch-chemische karakterisering te verbeteren. De pure PCL-steiger had bijvoorbeeld een treksterkte van 1,61 MPa, een rek van 122% en een Young's modulus van 7,01 MPa. De toevoeging van GO (2%) resulteerde in een aanzienlijke toename van de treksterkte tot 3,50 MPa, rek tot 131% en Young's modulus tot 15,15 MPa [80].
Gestimuleerd door het succes van het gebruik van grafeenfamiliematerialen als versterkingsmateriaal, combineerden veel teams de biocompatibiliteit van synthetische of biopolymeren met opmerkelijke fysieke eigenschappen van grafeenfamiliematerialen. Ze verwachtten een ideale composietsteiger te bereiken met verbeterde mechanische eigenschappen, geschikte porositeit, structurele ontwerpen en uitstekende biocompatibiliteit, om nieuwe botvorming te ondersteunen en te induceren.
Grafeenfamilie met op calciumfosfaat gebaseerde materialen
Menselijk bot bestaat uit 30% organisch materiaal, voornamelijk collageen, en 70% anorganisch materiaal, voornamelijk hydroxyapatiet (HA; Ca10 (PO4 )6 (OH)2 ) [81, 82]. Synthetische materialen op basis van calciumfosfaat zoals HA, β-tricalciumfosfaat (β-TCP) en calciumfosfaatcementen (CPC) zijn populaire steigermaterialen vanwege hun vergelijkbare samenstelling en structuren als de natuurlijke minerale fase van bot en hun goede botvormende eigenschappen vaardigheden [83,84,85]. In het bijzonder, vanwege de goede osteoconductie en osteo-inductiecapaciteit van HA [86], wordt het lange tijd op grote schaal gebruikt als kunstmatige bottransplantaten in orthopedische of maxillofaciale chirurgie om gebieden met botdefecten te herstellen [11, 71]. De inherente nadelen van HA-materiaal moeten echter worden verbeterd, zoals moeilijkheden bij het vormen, eigenaardige brosheid en lage breuktaaiheid [87, 88]. Er werd gemeld dat materiaalversterkte HA-composieten uit de grafeenfamilie werden ontwikkeld en aanzienlijk verbeterde breuktaaiheid en biologische prestaties. Zo werden HA/grafeencomposieten bereid door middel van vonkplasma-sintering (SPS), wat een aanvaardbare sterkte van HA opleverde [89]. Raucci et al. gecombineerd HA met GO in twee verschillende benaderingen:in situ sol-gel benadering en biomimetische benadering. De HA-GO verkregen door in situ sol-gel-benadering verbeterde de cellevensvatbaarheid van hMSC's en induceerde osteoblastische differentiatie zonder osteogene factoren te gebruiken. De HA-GO gevormd via biomimetische benadering hield de levensvatbaarheid en proliferatie van de cellen aan [90]. Bovendien kan het gereduceerde grafeenoxide (rGO) ook worden gebruikt als versterkingsmateriaal voor HA. De breuktaaiheid van de HA-rGO-composieten bereikte 3,94 MPa m 1/2 , een toename van 203% in vergelijking met pure HA. De HA-rGO verbeterde de celproliferatie en osteoblastische differentiatie, die werd beoordeeld door alkalische fosfatase (ALP) -activiteit van de menselijke osteoblastcellen [91]. Daarnaast hebben Nie et al. succesvol gesynthetiseerd rGO en nano-hydroxyapatiet (nHA) 3D poreuze composieten steiger (nHA@rGO) via zelfassemblage. De GO-oplossing vermengd met nHA-watersuspensie die werd verwarmd om het zelfassemblageproces te induceren. Ten slotte werden de reactieproducten gevriesdroogd om de 3D poreuze steiger te verkrijgen. De nHA@rGO-steiger kan de celproliferatie, ALP-activiteit en osteogene genexpressie van mesenchymale stamcellen (rBMSC's) van rattenbot aanzienlijk vergemakkelijken. En in vivo experiment verduidelijkte dat 20% nHA-geïntegreerde rGO (nHA@rGO) poreuze steiger de genezing van de circulaire calvariale defecten bij konijnen kan versnellen [92]. Bovendien hadden niet alleen dubbele componenten, maar ook driecomponenten uitstekende prestaties met goede cytocompatibiliteit en verbeterde hydrofiele en mechanische eigenschappen [93,94,95].
Tricalciumfosfaat, analoog van calciumfosfaat, is een tertiair calciumfosfaat, ook bekend als botas [Ca3 (PO4 )2 ]. Het dient als een overvloedige bron voor calcium en fosfor, die gemakkelijk kunnen worden opgenomen. Beta-tricalciumfosfaat (β-TCP) is zeer biocompatibel en creëert een resorbeerbaar in elkaar grijpend netwerk binnen de defectlocatie om genezing te bevorderen [96]. Wu et al. heeft met succes 2D β-TCP-GO-schijven en 3D β-TCP-GO-steigers gesynthetiseerd. Vergeleken met β-TCP en blanco controle, verbeterden de 2D β-TCP-GO-schijven de proliferatie, ALP-activiteit en osteogene genexpressie van hBMSC's aanzienlijk door de Wnt-gerelateerde signaalroute te activeren, wat wijst op de uitstekende in vitro osteostimulatie-eigenschap van GO- gemodificeerde β-TCP [85]. Het is bekend dat de Wnt-canonieke signaalroute een niet-triviale rol speelt bij het reguleren van cellulaire activiteiten zoals celproliferatie, differentiatie en morfogenese [97, 98]. In vivo onderzoek toonde aan dat 3D β-TCP-GO-steigers een grotere vorming van nieuw bot hadden in de calvariale defecten dan pure TCP-steiger (Fig. 3) [85]. Een nieuwe scaffold, calciumfosfaatcement opgenomen GO-Cu nanocomposieten scaffolds (CPC/GO-Cu) vergemakkelijkte de adhesie en osteogene differentiatie van rBMSC's, waarvan werd bevestigd dat ze de expressie van Hif-1α in rBMSC's kunnen opreguleren door de Erk1 / 2 signaalroute en induceerde de secretie van vasculaire endotheliale groeifactor (VEGF) en BMP-2-eiwit. Verder werden de CPC/GO-Cu-steigers getransplanteerd in ratten met calvariale defecten van kritische grootte en de resultaten toonden aan dat de steigers (CPC/GO-Cu) de angiogenese en osteogenese in de defecte gebieden significant bevorderden [99].
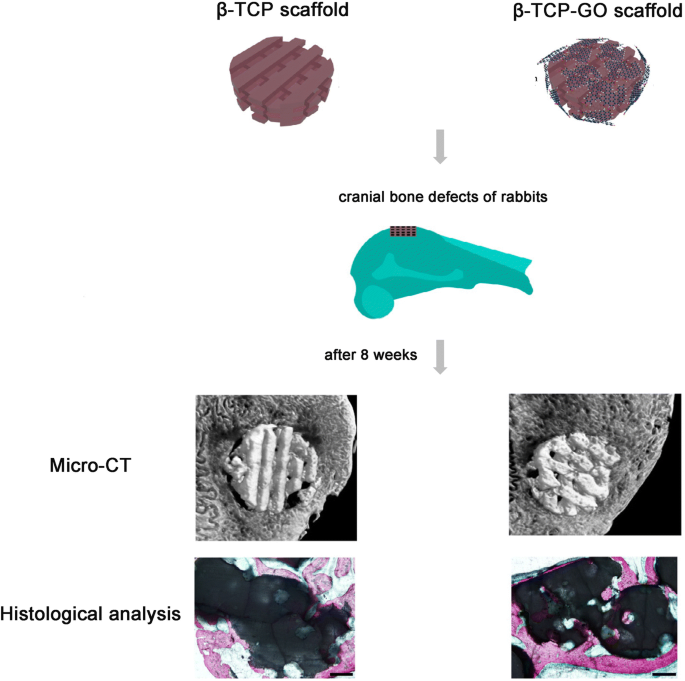
Schemaillustratie voor β-TCP- en β-TCP-GO-steigers stimuleerde de in vivo osteogenese. Micro-CT-analyse en histologische analyse van in vivo botvormingsvermogen voor de β-TCP- en β-TCP-GO-steigers na geïmplanteerd in de schedelbotdefecten van konijnen gedurende 8 weken. Overgenomen van ref. [85] met toestemming van de Journal of Carbon
Grafeenfamilie met Chitosan
Chitosan (CS), a highly versatile biopolymer, derived from the shells of crustaceans [1, 87], has a hydrophilic surface that promotes cell adhesion and proliferation and its degradation products are nontoxic. Chitosan is biocompatible, osteoconductive, hemostatic, and can be easily converted into the desired shapes [2]. Besides, chitosan can promote bone matrix of mineralization [1] and minimize the inflammatory response after implantation [100]. All properties above make chitosan especially attractive as a bone scaffold material. But the most challenging part is the obtainment of CS-based scaffolds with good mechanical properties and processability [101]. Interestingly, CS/GO scaffolds have high water-retention ability, porosity, and hydrophilic nature [101, 102]. The CS-based 3D materials were enriched with GO in different proportions (0.5 wt% and 3 wt%). The new developed CS/GO 3 wt% scaffold was expected to be ideally designed for bone tissue engineering applications in terms of biocompatibility and properties to promote cell growth and proliferation [103]. Another CHT/GO scaffold with 0, 0.5, and 3 wt.% GO were prepared by freeze-drying method. Similarly, the CS/GO 3 wt% scaffolds significantly enhanced the ALP activity in vitro and the new bone formation in vivo, suggesting a positive contribution of 3 wt% GO to the efficiency of osteogenic differentiation process (Fig. 4) [3]. All results proved that CS/GO scaffolds could be a feasible tool for the regeneration of bone defects, and the addition of a 3 wt% of GO to material composition could have a better impact on cell osteogenic differentiation.
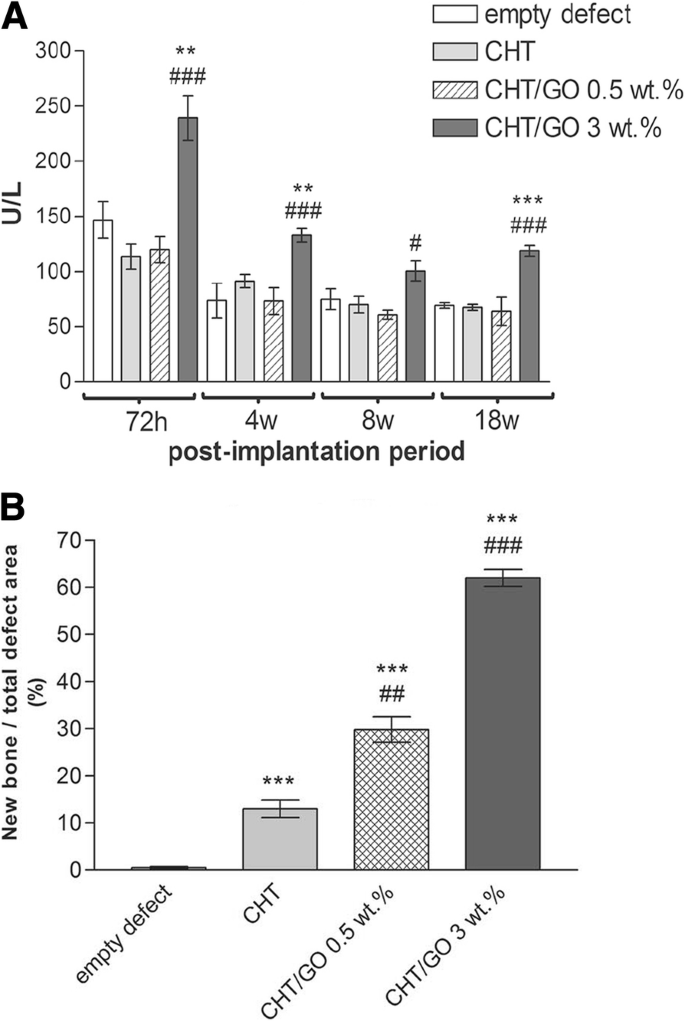
een ALP activity in mice calvaria defects implanted with CHT/GO and b histomophometric analysis of Masson Goldner trichrome-stained sections. ###p < 0.001 vs CHT; **p < 0.01 vs control; ***p < 0.001 vs control. Reproduced from ref. [3] with permission from the Journal of Scientific Reports
Moreover, some tricomponent composites, such as CS, GO, and HA can release more Ca and P ions compared to the pure HA nanoparticles, displaying a high bioactivity of the composite scaffold [87]. Ravichandran et al. fabricated a unique composite scaffold, GO–CS–HA scaffold, and the incorporation of GO enhanced the tensile strength of CS up to 8.2 MPa and CS–HA to 10 MPa. And the results demonstrated that GO–CS–HA scaffolds facilitated cell adhesion and proliferation, meanwhile showed improved osteogenesis in in vitro tests [2]. Another tricomponent composite scaffold, containing CS, gelatin (Gn), and different concentrates of graphene oxide (0.1%, 0.25%, 0.5%, and 1% (w /v ) GO) showed better physic-chemical properties than CS/Gn scaffolds. The addition of GO at the concentration of 0.25% to CS/Gn scaffolds exhibited enhanced absorption of proteins, extensive apatite deposition. The 0.25% GO/CS/Gn scaffolds were cyto-friendly to rat osteoprogenitor cells, and they enhanced differentiation of mouse mesenchymal stem cells into osteoblasts in vitro (Fig. 5). The tibial bone defect filled with 0.25% GO/CS/Gn scaffolds showed the growth of new bone and bridging the defect area, indicating their biocompatible and osteogenic nature [104]. Thus, no matter bicomponent or tricomponent composites scaffolds, the addition of graphene family materials to chitosan can favorably improve the mechanical properties and regulate the biological response of osteoblasts, promoting osteogenic differentiation.
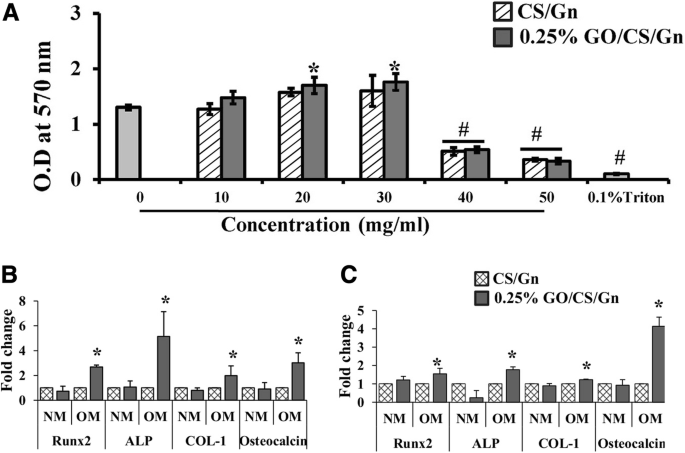
een MTT assay after incubation of CS/Gn scaffolds and 0.25% GO/CS/Gn scaffolds with media for 48 h. The asterisk indicates a significant increase versus control, and the pound sign indicates a significant decrease versus control (p < 0.05). b , c Expression of osteogenic-related genes (RUNX2, ALP, COL-1, and OC) in mMSCs cultured on CS/Gn scaffolds and 0.25% GO/CS/Gn scaffolds for 7 and 14 days measured by quantitative RT-PCR. Reproduced from ref. [104] with permission from the Journal of International Journal of Biological Macromolecules
Graphene Family with Other Synthetic or Bio-polymers
Sponge scaffolds of type I collagen, the major organic component of bone [81], have been clinically applied as scaffolds to regenerate bone tissue [105, 106]. Because collagen scaffolds (elastic moduli:14.6 ± 2.8 kPa) are relatively soft, the combination with GO is expected to enhance the elastic modulus of collagen scaffolds and to improve the osteogenic differentiation of MSCs for bone regeneration. The covalent conjugation of GO flakes to 3D collagen scaffolds (elastic moduli:38.7 ± 2.8 kPa) increased the scaffold stiffness by threefold and did not negatively affect the viability of BMSCs. The enhanced osteogenic differentiation observed on the stiffer scaffolds were likely mediated by BMSCs mechanosensing because the molecules involved in cell adhesion to stiff substrates were either upregulated or activated [107]. Moreover, the development of new biomaterials utilizing graphene family materials with high osteogenic capacity is urgently pursued (Table 2).
Up to now, these improved tricomponent systems for bone tissue engineering scaffolds possess good biocompatibility, which can promote cell attachment, proliferation, and have been reported mechanical properties matchable to those of natural bone. But the response to specific biological signals expressing, as well as the capabilities of enhancing cell differentiation and finally bone tissue regeneration, still needs to be explored further. Moreover, it has been reported that the pore structure (pore size, pore morphology, and pore orientation) and the elasticity of scaffolds were manipulated to regulate osteogenesis [108,109,110]. However, due to the complicated structure of porous and different elasticity accurately controlled of the scaffolds, it remains a major challenge to individually design specific pore architectures and elasticity 3D porous scaffolds that can stimulate bone regeneration. With the rapidly development of the science and technology, the emerging of the 3D-printing method may overcome this problem and open an avenue for bone tissue regeneration [85]. The in vitro bioactivity and excellent in vivo bone-forming ability of graphene family nanomaterials present a new prospect of developing a broad new type of multifunctional scaffolds for biomedical applications. Thus, we believe that the unraveled the molecular mechanisms behind will be revealed soon and graphene family materials still have attractive potential of applications in bone regeneration waiting us to explore.
Graphene Family Materials as Coating
Graphene family materials have been widely applied in diverse forms of medical applications for bone regeneration. As a coating, graphene family materials can be transferred on two dimensional (2D) flat non-metal or metal substrates to induce spontaneous osteogenic differentiation of several types of mesenchymal stem cells (MSCs) [64]. Nayak et al. transferred graphene to four 2D non-metal substrates (polydimethylsiloxane (PDMS), polyethylene terephthalate (PET), glass slide, and silicon wafer with 300 nm SiO2 (Si/SiO2 ).) and investigated the influence of graphene on BMSCs differentiation. They summarized that the graphene coating was cytocompatible and contributed to enhance the osteogenic differentiation of BMSCs at a rate comparable to differentiation under the influence of BMP-2 in the osteogenic medium [20]. Similarly, Elkhenany et al. found that goat BMSCs, seeded on 2D graphene-coated plates underwent osteoblastic differentiation in culture medium without the addition of any specific growth factors [8]. Simultaneously, Lee et al. tried to explain the origin of how graphene coating could accelerate stem cell renewal and differentiation. They deemed that the strong noncovalent binding abilities of graphene allowed it to serve as a preconcentration platform for osteoblastic inducers, which facilitated BMSCs osteogenic differentiation [67]. The capability of graphene in modulating osteogenic differentiation is evident. How about its derivatives? GO coatings and rGO coatings all showed favorable cytocompatibility and enhanced spontaneous osteogenic differentiation by upregulating levels of ALP activity [111, 112].
Since titanium (Ti) and medical-grade Ti alloy have been extendedly applied in the orthopedic and dental fields [113,114,115], satisfactory osseointegration for titanium and its alloys is still a major challenge and need to be explored deeply in order to help the clinicians to promote the success or survive rate of implants and diminish the likely complications encountered after their placement [114, 116, 117]. Graphene family materials coated titanium and its alloys, serving as a new method to improve their capabilities of osseointegration at the tissue-implant interface, attracted widespread attention. For example, GO-coated titanium enhanced cell proliferation, upregulated levels of ALP activity and gene expression level of osteogenesis-related markers, and promoted the protein expression of BSP, Runx2, and OCN [117]. Qiu et al. made different thickness GO coatings on the pure titanium surfaces respectively by cathodal electrophoretic deposition. Interestingly, with the increasing thickness of GO, the ALP-positive areas improved, ECM mineralization increased [118]. Moreover, Zeng et al. firstly fabricated GO/HA composite coatings by electrochemical deposition technique on Ti substrate. The addition of GO facilitated both the crystallinity of deposited apatite particles and the bonding strength of the as-synthesized composite coatings [119]. It is well known that hydrophilic surface is biocompatible compared to hydrophobic surface. In the case of rGO coating, the rapid adsorption of serum protein improves hydrophilia of graphene surface and enhances cell adhesion. Jia et al. used evaporation-assisted electrostatic assembly and one-pot assembly to fabricate 2D GO-coated Ti and rGO-coated Ti, with tailored sheet size and surface properties. Compared to the contact angle of titanium (60.4°), the contact angle of GO-coated Ti and rGO-coated Ti were 20° and 14.2°, respectively, indicating the successful interfacial assembly of graphene and excellent wettability properties. The rGO-coated Ti elicited better cell adhesion and growth than bulk GO, while the latter evoked higher activity of osteogenic differentiation [120].
Osseointegration is a complicated biological process determined by the surface properties of implants [114]. The graphene-based coatings above all lack 3D morphology. The 3D porous surface structure of coating can mimic the special macrostructures of the nature bone tissues [115]. Qiu et al. first synthesized 3D porous graphene-based coating on the pure titanium plates (GO@Ti and rGO@Ti). Water contact angles showed super hydrophilic surfaces of GO@Ti and rGO@Ti. Surface wettability exerts great effect on the biocompatibility of materials, which is strongly related to biomolecules adsorption [121]. GO@Ti and rGO@Ti both showed the excellent cytocompability and the optimal capability of osteoinduction [39]. Morin and his co-workers even transferred single or double chemical vapor deposition (CVD) grown graphene coatings onto 3D objects with differences in 3D geometries and surface roughness, such us dental implant, locking compression plate and mandible plate (Fig. 6) [64]. CVD is a very stable coating fabrication method, with substrate-independent properties and versatile surface functionalization. Besides, surface active CVD coatings are good platforms for immobilizing biomolecules, which is very important to bone regeneration [122].
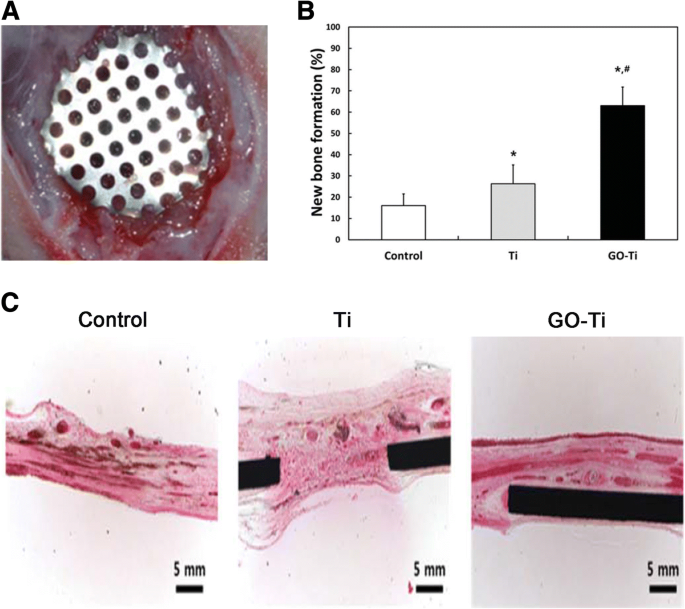
een The calvarial defects of rats were enclosed with a GO-Ti membrane. b New bone formation of the rat calvarial defects after the implantation of Ti or GO-Ti membrane at postoperative week 8. *p < 0.05 vs control; #p < 0.05 vs Ti. c Images of HE staining of the rat calvarial defects after the implantation of Ti or GO-Ti membrane at postoperative week 8. Reproduced from ref. [128] with permission from the Journal of Applied Spectroscopy Reviews
Overall, the strategy of applying graphene family materials as coating onto a surface is charming. Through currently available techniques or methods, such as CVD [123], electrochemical deposition [119], with diverse substrates (e.g. polymers, metals), graphene, and its derivatives can be obtained efficiently, with dimensions ranging from nanometer to macroscopic scales [120]. Then, graphene family nanomaterials can be transferred onto the substrate, either as 2D coatings/films/sheets or 3D porous structures of coating, to enable the binding of biomolecules, absorb the serum protein, and facilitate osteogenic differentiation of stem cells. But the different physical and chemical properties of the substrates and the type or frequent use of chemical inducers for osteogenic differentiation (e.g., dexamethasone, bone morphogenetic protein-2) that may cover up the effects exerted by graphene family materials alone [65]. Therefore, these methods still require to be well-directly improved and further studied.
Graphene Family as an Additive in Guided Bone Membrane
Barrier membranes are standardly used in oral surgical procedures, applying in guided tissue regeneration (GTR) and guided bone regeneration (GBR), for the treatment of periodontal bone defects and peri-implant defects, as well as for bone augmentation [124, 125]. GBR is considered to be one of the most promising methods for bone tissue regeneration. The concept of GBR is using a non-resorbable or absorbable membrane serving as a barrier to prevent the ingrowth of soft connective tissue into the bone defect and offer a space to “guide” the bone reconstruction [126, 127]. An ideal GBR membrane should have excellent biocompatibility and mechanical property to promote the regeneration of bone tissues and prevent soft-tissue ingrowth. Ti membrane is a non-resorbable membrane with excellent mechanical properties for the stabilization of bone grafts. Park et al. fabricated GO-coated Ti (GO-Ti) membranes, with increased roughness and higher hydrophilicity. GO endowed the pure Ti membranes better biocompatibility and enhanced the attachment, proliferation, and osteogenesis of MC3T3-E1 in vitro. Moreover, GO-Ti membranes were implanted into rat calvarial defects (Fig. 6) and new bone formation significantly in full-thickness calvarial defects without inflammatory responses was observed [128].
However, non-resorbable membranes need to be removed by a second operation. Thus, a resorbable membrane is recommended owing to avoid a second intervention during operation, which can diminish the risk of infection and the loss of the regenerated bone. But the resorbable membranes made of collagen or chitosan usually has poor mechanical property. The addition of graphene family materials improves the weaknesses of resorbable membrane. For instance, De et al. attempted to prepare absorbable collagen membranes enriched with different concentrations of GO. The presence of GO on the membrane altered the mechanical features of the membrane, by conferring lower deformability, improving stiffness, and increasing roughness [129]. Tian et al. made 3D rGO (3D-rGO) porous films, which can accelerate cell viability and proliferation, as well as significantly enhanced ALP activity and osteogenic-related gene expressions [130].
Although pristine graphene is basically incompatible with organic polymer to form homogeneous composite, and even decrease the cell viability in some cases if the amount of graphene is excessive [131]. The incorporation of graphene family materials can enhance the bioactivity and mechanical properties of composite membranes. Because of the potent effects on altering mechanical drawbacks, stimulating osteogenic differentiation, and exhibiting superior bioactivity, graphene family material-modified membranes can be applied effectively to GBR.
Graphene Family Materials as Drug Delivery System (DDS)
Due to their small size, intrinsic optical properties, large specific surface area, low cost, and useful noncovalent interactions with aromatic drug molecules, graphene family materials exhibit excellent efficacy as delivery vehicles of genes and biomolecules. Moreover, simple physisorption via π-π stacking, hydrogen bonding, and electrostatic interaction is able to assist in high drug loading of hydrophobic drugs without compromising potency or efficiency [38]. The therapeutic efficacy of drugs is always related to the drug delivery carrier, which should enable the loading of large doses, controlled release, and retention of the bioactivity of the therapeutic proteins [132]. At present, anticancer drugs, including doxorubicin [133,134,135,136,137], paclitaxel [138, 139], cisplatin [140], and methotrexate [141, 142] loaded by graphene family nanomaterials showed amazing cancerous effect for the selective killing of cancer cells.
For better bone regeneration, we sometimes need the help of osteogenic drug or macromolecular osteogenic protein. It was reported that the adsorbed drugs or loaded growth factors on graphene or its derivatives could enhance the osteogenic differentiation of cells due to the increased local concentration [143]. For example, simvastatin (SIM) chosen as a model drug was loaded on the 3D porous scaffolds, which were made of silk fibroin (SF) and GO. SIM is an inhibitor of the competitive 3-hydroxy-3-methyl coenzyme A (HMG-CoA) reductase [144]. The effects of SIM on bone formation are associated with an increase in the expression of bone morphogenetic protein-2 (BMP-2) mRNA and enhanced the vascular endothelial growth factor (VEGF) expression [145, 146]. SIM can release sustainedly (30 days), and the release rate was relevant to the GO content within the scaffolds. In vitro, compared with the blank scaffolds, the SF/GO/SIM showed better biocompatibility, and the cells cultured on them exhibited faster proliferation rate [147]. Dexamethasone (DEX) is an osteogenic drug for which can facilitate osseointegration. Jung et al. firstly loaded DEX on rGO-coated Ti by π-π stacking. The loading efficiency of DEX on rGO-Ti was 31% after drug loading for 24 h and only 10% of total loaded DEX was released for 7 days, indicating that the drug delivery system can induce a long-term stimulation of stem cells for osteogenic differentiation. The DEX/rGO-Ti significantly facilitated MC3T3-E1 cells growth and differentiation into osteoblasts [143]. Similarly, Ren et al. also employed the GO-Ti and rGO-Ti as drug vehicles to absorb DEX. The presence of DEX-GO and DEX-rGO helped to promote the cell proliferation and largely enhanced osteogenic differentiation [115]. The graphene family materials coating on Ti alloys with controlled drug delivery can stimulate and enhance cellular response around implant surface to reduce the osseointegration time, expected to be applied for various dental and biomedical applications [143].
Not only small molecular osteogenic drug, but also macromolecular proteins can be loaded by graphene family materials for bone regeneration. Bone morphogenetic proteins (BMPs) are the most potent osteoinductive protein for bone regeneration. Thus, BMP-2 was loaded on the surface of Ti/GO through π-π stacking and the interaction between negatively charged carboxylic groups at the edges of GO and positively charged amino acid residues of BMP-2 [132]. Ti/GO/BMP-2 exhibited the high loading and the sustained release of BMP-2 with preservation of its 3D conformational stability and bioactivity. In vitro, the capability of Ti/GO/BMP-2 is to enhance osteogenic differentiation of hBMSCs. In a mouse calvarial defect model, compared to Ti/BMP-2 implants, Ti/GO/BMP-2 implants around had much more extensive bone formation [132]. Xie et al. used GO-modified hydroxyapatite (HA) and GO-modified tricalcium phosphate (TCP) as an anchor for adsorbing BMP-encapsulated BSA- nanoparticles (NPs) respectively. The charge balance and BMP-2 sustained release capability of the new scaffolds synergistically improved BMSCs proliferation, differentiation, and bone regeneration in vivo [148]. Poor osteointegration and infection are the most serious complications leading to failures of Ti implantation [10]. Han et al. incorporated GO onto polydopamine (PDA)-modified Ti scaffolds. Then, BMP-2 and vancomycin (Van) were separately encapsulated into gelatin microspheres (GelMS). After that, drug-containing GelMS were loaded on GO/Ti scaffolds and anchored by the functional groups of GO (Fig. 7). The new scaffolds were endowed with dual functions of inducing bone regeneration and preventing bacterial infection [149]. Substance P (SP) is a highly conserved 11 amino acid neuropeptide [150], involved in many processes, such as the regulation of inflammation, wound healing, and angiogenesis, and it is expected to promote MSC recruitment to the implants [151]. Therefore, apart from BMP-2, La et al. added this peptide, SP, on the surface of GO-coated Ti. The dual delivery system via GO-coated Ti showed sustained release of BMP-2 and SP and the potential of SP for inducing migration of MSCs. In vivo, Ti/GO/SP/BMP-2 group showed the greater new bone formation in the mouse calvaria than Ti/GO/BMP-2 group may be due to the MSCs recruitment by SP to the implants [152].
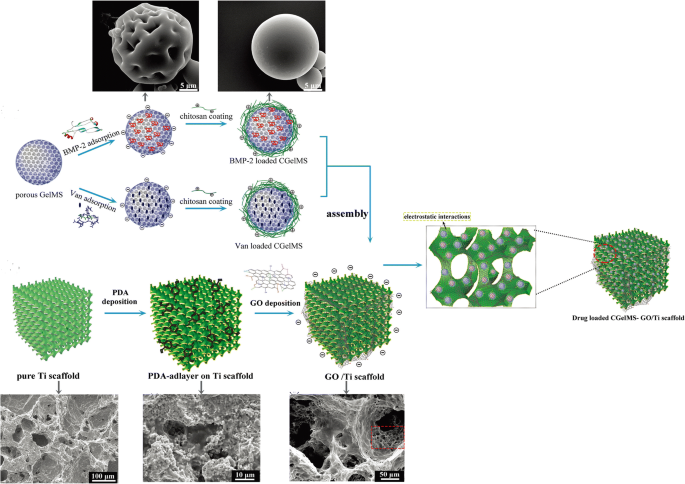
Schematics and scanning electron micrographs of the preparation the new GO/Ti scaffold:BMP2- and Van-loaded CGelMS were immobilized on the GO/Ti scaffold through electrostatic interactions between the functional groups of GO and CGelMS. Reproduced from ref. [149] with permission from the Journal of Biomaterials Science
Currently, more and more teams get down to designing new drug delivery system to improve the practical applications. The loading of large doses, controlled release, and retention of the bioactivity of the therapeutic proteins are still difficulty in research on drug delivery system.
Conclusies
Studies on the graphene family materials on biological applications is emerging rapidly, especially their potential applications for bacteria inhibition and inducing stem cell osteogenic differentiation. Before their biological applications are considered for clinical trial, the biocompatibility of graphene family materials is of vital importance. However, the challenges exist and must be overcome. These challenges include a thorough understanding of the graphene-cell (or tissue, organ) interaction and cellular uptake mechanism as well as mechanism(s) of potential toxicity. We summarize and analyze several articles and conclude that the cytotoxicity and in vivo biocompatibility of graphene family materials are influenced by numerous factors, including surface functionalization, concentration, size, and shape. At low concentration, graphene family materials are cytocompatible, with little negative influence on cell morphology, viability, and proliferation. Furthermore, it was reported that graphene family materials with flat shapes having better biocompatibility, because the flat shape materials were expected to have minor interaction with the cellular membranes [47]. Although the different criteria were used to define the size scale and shape of graphene and its derivatives, it was true that nano-sized graphene family materials were much safer for biomedical applications [54]. Size-control synthesis of graphene family materials needs to be considered prudently in subsequent researches. Moreover, the major challenge for researchers lies in understanding how graphene family materials behave in complicated microenvironment and establishing the long-term biocompatibility of graphene and its derivatives. Thus, researchers should spare no efforts to keeping studying the bio-safety of graphene family materials in vivo, as well as in vitro, to further understand the intricate interaction between cells and the materials. Although some papers raise concerns about bio-safety, after better control of the modifying of graphene family materials during synthesis, the potential versatility that graphene family uniquely offers has made it a competitive candidate of option for biomedical applications.
On the one hand, a lot of researches have pointed out that graphene family materials possess the capability of bacteria inhibition, due to their functional chemical groups, sharp edges, and synergistic effect with other drugs. Besides, bone remolding and regenerating successfully in an infective bone defect area is challenging. Peri-implant infection and poor osseointegration are also major challenges we confront. The use of graphene family materials in the design and development of antimicrobial bone regeneration application will capture tremendous attention in the future.
On the other hand, lots of teams painstakingly did researches to design and fabricate the new strategies of applying graphene family materials in bone tissue engineering. 3D graphene-based scaffold is a promising biocompatible scaffold, which can enhance pre-osteoblasts or stem cells osteoblastic differentiation. Graphene family materials also can be added as a reinforced material aiming to strengthen the composite scaffold mechanical properties and improve physicochemical characterization. In addition, the strategy of applying graphene or its derivatives as coating onto a surface is charming, which is expected to possess the antibacterial activity and better osseointegration, especially the 3D coating. It has been generally hypothesized that the surface characteristics of graphene family materials including nanostructures, surface roughness, protein absorption ability, electrostatic interactions, and surface hydrophilicity, exert an enormous effect on the molecular pathways which control the fate of stem cells [39, 115]. The 3D structure of scaffold or coating allows nutrients to be freely delivered, which influences the biocompatibility of the graphene family. But the manufacturing method of 3D scaffold or coating is relatively difficult and complicated. However, with the rapidly development of the science and technology, the emerging of the 3D-printing method may overcome this problem and open an avenue for bone tissue regeneration.
Moreover, graphene family materials show great potential in GBR and DDS as well. Graphene family materials improve poor mechanical property of the resorbable membranes made of collagen or chitosan without compromising their intrinsic property. Osteogenic drug or macromolecular osteogenic protein can be adsorbed on graphene or its derivatives via π-π stacking, hydrogen bonding, and electrostatic interaction with high loading and good efficiency. Taking the varied merits into consideration, graphene family materials hold great potential to bone tissue regeneration.
Considering that many supreme properties graphene and its derivatives have, especially in vitro osteogenesis enhancing ability and excellent in vivo bone-forming ability, although they still have drawbacks, graphene family materials still are promising candidates used for bone regeneration applications.
Afkortingen
- ALP:
-
Alkaline phosphatase
- BMP-2:
-
Bone morphogenetic protein-2
- BMSC:
-
Bone mesenchymal stem cells
- CPC:
-
Calcium phosphate cements
- CVD:
-
Chemische dampafzetting
- CXYG:
-
Carboxyl graphene
- DEX:
-
Dexamethasone
- ECM:
-
Extracellulaire matrix
- GBR:
-
Guided bone regeneration
- GelMS:
-
Gelatin microspheres
- GNOs:
-
Graphene nano-onions
- GO:
-
Grafeenoxide
- GONPs:
-
Graphene oxide nanoplatelets
- GONRs:
-
Graphene oxide nanoribbons
- GQDs:
-
Graphene quantum dots
- HA:
-
Hydroxyapatite
- HUVEC:
-
Human umbilical vein endothelial cells
- MC3T3-E1:
-
A murine pre-osteoblastic cell line
- MWNTs:
-
Meerwandige koolstofnanobuisjes
- OPE:
-
Oxygen plasma etching
- PCL:
-
Polycaprolactone
- PDA:
-
Polydopamine
- PDLLA:
-
Poly (d, l-lactic acid)
- PDLSC:
-
Periodontal ligament stem cells
- PDMS:
-
Polydimethylsiloxane
- PEG:
-
Polyethyleenglycol
- PET:
-
Polyethylene terephthalate
- PLA:
-
Poly-lactic acid
- PLGA:
-
Poly-glycolic acid
- PPY:
-
Polypyrrool
- rGO:
-
Gereduceerd grafeenoxide
- ROS:
-
Reactieve zuurstofsoorten
- SIM:
-
Simvastatin
- SP:
-
Substance P
- SPS:
-
Spark plasma sintering
- Ti:
-
Titanium
- Van:
-
Vancomycin
- VEGF:
-
Vascular endothelial growth factor
- β-TCP:
-
β-Tricalcium phosphate
Nanomaterialen
- 13 soorten vuurvaste materialen en hun toepassingen
- Intern onderzoek en ontwikkeling
- Grafeen in luidsprekers en oortelefoons
- Groen en duurzaam:milieuvriendelijke composietmaterialen
- IIoT-trends en uitdagingen om te bekijken
- Nanodeeltjes voor kankertherapie:huidige vooruitgang en uitdagingen
- Vooruitgang en uitdagingen van fluorescerende nanomaterialen voor synthese en biomedische toepassingen
- Grafeen- en polymeercomposieten voor toepassingen met supercondensatoren:een recensie
- Op weg naar TiO2-nanovloeistoffen - Deel 2:Toepassingen en uitdagingen
- Bioveiligheid en antibacterieel vermogen van grafeen en grafeenoxide in vitro en in vivo
- Materialen en constructie van flexibele circuits